Salvatore Di Maio1, Pierluigi Marzuillo2, Demetris Mariannis3, Soteroula Christou4, Andreas Ellinides5, Costantinos Christodoulides6 and Vincenzo de Sanctis7.
1 Emeritus Director in Pediatrics, Santobono-Pausilipon Children's Hospital, Naples, Italy.
2 Department of Woman, Child, General and Specialized Surgery, University "Luigi Vanvitelli", Naples. Italy.
3 Royal Lancaster Infirmary, Lancaster, Lancashire, United Kingdom.
4 Thalassemia Unit, Nicosia, Cyprus.
5 Medical Student, European University Cyprus, School of Medicine, Nicosia, Cyprus.
6 Pediatric Registrar, Archbishop Makarios III Hospital, Nicosia, Cyprus.
7 Pediatric and Adolescent Outpatient Clinic, Quisisana Hospital, Ferrara, Italy.
Correspondence to: Vincenzo de Sanctis, MD.Coordinator of the
International Network of Clinicians for Endocrinopathies in Thalassemia
and Adolescence Medicine (ICET-A) and Pediatric and Adolescent
Outpatient Clinic, Quisisana Hospital, Ferrara, Italy. Via Paolo V, 25.
44121 Ferrara, Italy. Tel: +39 0532 770243. E-mail:
vdesanctis@libero.it
Published: July 1, 2021
Received: March 3, 2021
Accepted: June 4, 2021
Mediterr J Hematol Infect Dis 2021, 13(1): e2021040 DOI
10.4084/MJHID.2021.040
This is an Open Access article distributed
under the terms of the Creative Commons Attribution License
(https://creativecommons.org/licenses/by-nc/4.0),
which permits unrestricted use, distribution, and reproduction in any
medium, provided the original work is properly cited.
|
Abstract
Background:
Menarche is an important milestone in a feminine reproductive life, and
regular menstrual cycles reflect normal functioning of the
hypothalamic-pituitary-ovarian axis, a vital sign of women's general
health. Aim of the study:
We explored the age at menarche and the following menstrual cycles
characteristics among 85 unmarried Transfusion-Dependent β-Thalassemia
(TDT) women, born between 1965 and 1995, concerning iron chelation
therapy (ICT) with desferrioxamine (DFO) and nutritional status,
assessed by body mass index (BMI). Results:
53 adolescents who had begun ICT before the age of 10 years experienced
menarche at 13,7 ± 1,6 years (mean ± DS), whereas 32 who began
treatment after ten years experienced menarche significantly later
(15.5 ± 1.9 yrs; p: 0.001). At the age of menarche: BMI-Z score (n= 67,
- 0,09 ±1) was inversely correlated with both age at starting ICT (r =
- 0,39; p = 0001) and age at menarche ( - 0,45, p = 0,0001). Serum
ferritin levels (SF) were significantly correlated with the age at
starting chelation therapy (n = 79; r = 0,34; p = 0,022). In 56 TDT
adolescents who developed secondary amenorrhea (SA), the SF levels were
significantly higher (4,098 ± 1,907 ng/mL) compared to 23 TDT
adolescents with regular menstrual cycles (2,913±782 ng/mL; p = 0,005).
Nutritional status of "thinness" at menarche was associated with a
lower prevalence of subsequent regular menstrual cycles and a higher
prevalence of early SA. Conclusion:
An early ICT in TDT patients was associated with a normal "tempo" of
pubertal onset and a higher frequency of subsequent regular menstrual
cycles. In TDT patients, who developed SA, a diagnosis of acquired
central hypogonadism was made, mainly due to the chronic exposure to
iron overload, however other potential causes linked to nutritional
status, deficient levels of circulating nutrients, and the chronic
disease itself cannot be fully excluded.
|
Introduction
The
hemoglobinopathies (mainly thalassemias and sickle-cell disease) are
the most frequent inherited genetic disorders worldwide, with some
300,000 infants born annually with major hemoglobinopathies.
Approximately 80% of these births occur in low- and middle-income
countries where the control and management of hemoglobinopathies are
meager.[1] At present, thalassemia diseases are
classified into transfusion-dependent thalassemia (TDT) and
non-transfusion-dependent thalassemia (NTDT).[2]
Current treatment of TDT consists of regular transfusions that lead to
iron overload, requiring iron chelation to prevent iron-related organ
toxicity. Iron overload-related complications include involvement of
the heart, liver, and endocrine glands.[3]
Iron
accumulates at different rates in various organs, and these organs show
different susceptibility to the damage induced by reactive iron species
such as non-transferrin bound iron (NTBI) and the intracellular labile
iron pool (LIP).[4-6]
In clinical practice, serum
ferritin (SF) measurement and non-invasive imaging techniques are
available to diagnose iron overload, quantify its extent in different
organs, and monitor clinical response to therapy.
Three iron
chelators (ICT) are currently available to treat iron overload:
desferrioxamine (DFO), deferiprone (DFP) and deferasirox (DFX). DFO is
administered subcutaneously (s.c.) over 8–24 hours, 6–7 nights per
week, DFP and DFX are given orally as a monotherapy or in combination
with DFO.[7,8]
Overall, ICT results in better overall survival, primarily if it is instituted early and the compliance is maintained.
Menarche
is one of the markers of puberty and is considered an essential
milestone in a woman's reproductive life. Irregular and prolonged
menstrual cycles, often attributed to the functional disruption of the
hypothalamic-pituitary-ovarian axis, are, however, common among women
of reproductive age. They have been associated with a greater risk of
non-communicable diseases, including ovarian cancer, coronary heart
disease, type 2 diabetes, and mental health problems.[9]
Few
reports on the relationship between the age at the beginning of ICT and
age at menarche in patients with TDT are reported in the literature,[10] and practically nothing, to the best of our knowledge, of their menstrual history.
Our
retrospective study aimed to collect data on age at menarche, body mass
index (BMI), and the menstrual characteristics in a group of unmarried
Italian and Cypriot TDT women treated with s.c. DFO, given before and
after 10 years (yrs), from the 70s to 2000s.
Patients and Study Design
Clinical
data were obtained from medical records of 85 TDT adolescent girls,
followed from 1978 to 2018 at the Thalassemia Centers of Ferrara
(Italy) (72 patients) and Nicosia (Cyprus) (13 patients). In Ferrara,
the study was started by VDS in January 1977 and was completed in 2018,
by the same physician, at the Pediatric and Adolescent Outpatient
Quisisana Clinic of Ferrara (Italy).
Exclusion criteria were: 1)
NTDT; 2) mental illness (depression, anxiety disorders, eating
disorders, and addictive behaviors); 3) chronic kidney diseases; 4)
history of severe head injury; 5) bone marrow transplanted patients; 7)
HIV positivity; 8) TDT patients with poor or inconsistent control of
associated endocrine complications; 9) autoimmune diseases, chromosomal
abnormality, gonadotoxic treatment by systemic chemo- or radiation
therapy; 10) smoking of more than 15 cigarettes/day or alcohol abuse,
and 11) patients with a clinical menstrual history of isolated
menarche. The latter condition was reported in 10 TDT patients.
The
study was restricted to the analysis of the following available data:
patients' age at menarche (n = 85), menstrual characteristics (n = 79)
and persistence of menstrual cycles at the last clinical examination (n
= 23), age at starting ICT and duration of treatment (n = 85). In
addition, nutritional status, assessed by BMI, was available at
menarche in 20 patients with subsequent preserved menstrual cycles and
in 47 patients who developed secondary amenorrhea (SA), at the age of
SA in 61 patients and at last examination in 14 patients with preserved
menses.
Serum ferritin levels (SF) were available at menarche in
56 patients who developed SA and in 23 with preserved menstrual cycles,
at the age of SA in 56 patients, and at last examination in 14 with
preserved menstrual cycles. Therefore, the data of 55 patients with SA
were coupled with those registered at menarche.
The regular tempo
of menarche was defined arbitrarily as the time interval from 11 to 14
yrs of age, derived from the ± 1,25 standard deviations (SD) of the
average age of 12,4 ± 1,3 yrs of Italian and Greek population.[11,12] Menses were defined as regular[13]
if menstrual cycle length was between 21 and 35 days (45 days during
the first post-menarcheal year); irregular (oligomenorrhea) if the
menstrual interval was > 35 days and < 6 months. SA was defined
as the absence of menstruation for 6 months and over at any time after
menarche.[14] The number of "gynecologic years" was
considered the interval time between age at menarche and the duration
of menstrual history. It was determined in 23 patients with preserved
menstrual cycles up to the last examination and in 62 patients from
menarche to the age of SA.
BMI and BMI Z- Score were calculated with the formula weight in kg/ height in m2 (Kg/Ht m2) based on the Center for Disease Control (CDC) growth charts [http://zscore.research.chop.edu]. The BMI cut-off, by age and sex, was defined according to Cole et al.[15] Thus, BMI values between 18.5 and 25 (Kg/Ht m2)
were classified as normal, between 17 - 18.5 as mild thinness, between
16 - 17 as moderate thinness, < 16 as severe thinness and
overweight/obesity if > 25-30, and over (Kg/Ht m2).
Patients
with SA had been evaluated for pituitary–ovarian axis integrity
(luteinizing hormone–LH and follicle-stimulating hormone–FSH before and
after stimulation with gonadotropin-releasing hormone (Gn-RH
stimulation test), prolactin, and 17 β-estradiol. In addition, blood
samples were assayed for FSH and LH before and 20, 40, 60, and 120
minutes after injection.
A lack of gonadotropin release to the
Gn-RH test (1-fold to 1.5-fold increase from the baseline level)
associated with a negative medroxyprogesterone acetate test (MAP test:
10 mg given orally for 7 days), in the presence of a normal PRL and
TSH, was considered as an index of hypothalamic and/or pituitary SA. In
addition, hyperprolactinemia was defined by a high level of serum
prolactin (PRL) above the standard upper limit of the normal range.
Pelvic ultrasonography was performed to determine the pelvic organs' morphology and maturity and exclude pathologies.
The
patients' iron overload was assessed by SF. The manufacturer’s normal
reference range values in females were 15–150 ng/mL. Iron overload was
arbitrarily classified as mild (SF < 1,000 ng/mL), moderate (SF:
>1,000 ng/mL and < 2,000 ng/mL) or severe (SF: >2,000 ng/mL).[16]
Ethical
approval for the study was obtained in accordance with local
institutional requirements according to the Declaration of Helsinki [http://www.wma.net].
Statistical analysis.
Differences for continuous variables were analyzed with the
independent-sample t-test for normally distributed variables and with
the Mann-Whitney test in case of non-normality. Qualitative variables
were compared using the chi-squared test. Finally, a comparison of
regression lines by ANOVA was performed to examine the relationship
between different parameters. The Stat-Graph XVII software for Windows
was used for all statistical analyses. A p-value < 0.05 was
considered statistically significant.
Results
Clinical characteristics and menstrual history of TDT patients.
Before 1972, blood transfusions were given when anemia was severe
enough to cause symptoms. After that, patients were regularly
transfused every 2-3 weeks to maintain the mean pre-transfusional
hemoglobin (Hb) level around 9.5 g/dL. Treatment with intramuscular
DFO, at a dose of 20 mg/kg body weight (b.w.), was available for most
patients since 1969. Regular subcutaneous (s.c.) DFO infusion was
started in 1978 in patients older than 2 years. Initially, the
recommended DFO dose was 20 mg/kg body weight administered daily at
night by infusion pump over 10 hours. Based on transfusional iron input
the dose increased to 40 mg/kg body weight (b.w.) in 1982 and up to 60
mg/ kg b.w. in 1984. Ascorbic acid was added orally at a dose of 2-5
mg/kg (maximum dose 200 mg) in a selected group of patients.
Since
1995, the oral chelator DFP has been available; it was given at a dose
of 75 mg/kg/b.w. to some patients over 11 years, as monotherapy or
combined with DFO. In 2007, DFX was introduced at a dose of 25-30 mg/kg
b.w. for patients whose treatment with DFO was contraindicated or
inadequate.
Our patients' mean age at the start of chelation was
7,6 ± 3,8 yrs. Twenty-four of them started therapy before the age of 5
(2,8 ± 1,2 yrs).
At the last clinical examination in 2018, the
mean age of 85 TDT patients was 36,5 ± 5,7 years. Over the years, the
age at menarche ranged from 11 to 19,5 years (mean age of 14,3 ± 1,8
yrs). At last observation, 23 patients were still menstruating, whereas
62 (72,9 %) had lost the menstrual periods (SA), at a mean age of 18,0
± 3,5 yrs. In patients with preserved menstruations, the age at
menarche was lower (13,4 ± 0,8 yrs) than in patients who developed SA
(14,6 ± 2,0 yrs; p = 0,007). No statistical significant difference was
present between their mean chronological age: 33,8 ± 7,2 yrs versus
37,0 ± 5,3 yrs, respectively (p = 0,15).
The duration of
“gynecological years” of the whole group of subjects correlated
significantly with the years at birth (n = 85, r = 0,25; p = 0,02). At
the last clinical examination, the duration of "gynecological years" in
the 23 women with preserved menses was 21,2 ± 5,9 yrs, whereas in the
62 women who experienced SA was 3,5 ± 3,0 yrs (p = 0,0001).
BMI-Z
score at menarche was 0,5 ± 0,9 in the 23 patients with preserved
menstruations and – 0,32 ± 1,0 in 50 patients who developed SA (p =
0,002).
Iron chelation therapy (ICT) in relation to menstrual history.
At menarche, the mean SF level in 79 TDT patients was 3,753 ± 1,741
ng/mL. A significant correlation was present between the starting ICT
age and SF at menarche (Figure 1).
The subgroup of the 23 TDT patients with preserved menstrual cycles had
a mean SF level at menarche significantly lower compared to 55 patients
who developed SA (2,913 ± 782 ng/mL versus 4,098 ± 1,907 ng/mL; p =
0,005), although in the latter group of patients, the mean SF level at
SA was lower (3,163 ± 2,125 ng/mL) compared to the values registered at
menarche (4,098 ± 1,907 ng/mL; p = 0,01).
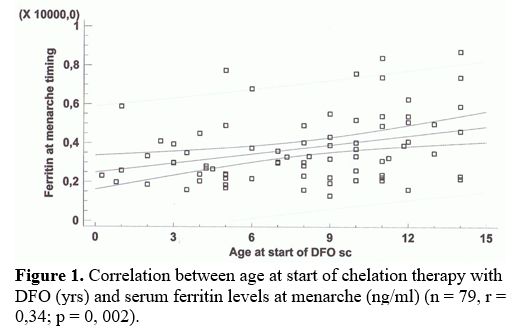 |
Figure
1. Correlation between age at start of chelation therapy with DFO (yrs)
and serum ferritin levels at menarche (ng/ml) (n = 79, r = 0,34; p = 0,
002).
|
Despite
an early iron chelation therapy (mean age: 2,8 ± 1,2 yrs), 11 out of 24
of our TDT patients developed a precocious SA, at a mean age of 19,5 ±
5,9 years with a mean SF level of 1,758 ± 846 ng/mL.
Finally, the
mean SF at last examination in 14 women with preserved menses, was
significantly lower (1,938 ± 837 ng/mL) compared to 55 TDT patients
with SA (3,163 ± 2,125 ng/mL; p = 0,03).
Menarcheal age in relation to ICT and BMI.
The patients' year of birth was inversely correlated with the age at
menarche (n = 85, r = ‒ 0,27; p: 0,01) and the age at start of ICT (n =
85, r = ‒ 0,86; p: 0,00001).
The TDT patients who had begun ICT
before the age of 10 years (n = 53) experienced menarche at 13,7 ± 1,6
yrs, whereas those who begun treatment after (n = 32), at a
significantly later age (15,5 ± 1,9 yrs; p: 0,001). Table 1
summarizes the distribution of patients' mean age at menarche (≤ or
> 14 years) in relation to the age at ICT start with DFO; the
frequencies were significantly different (χ² = 0,016).
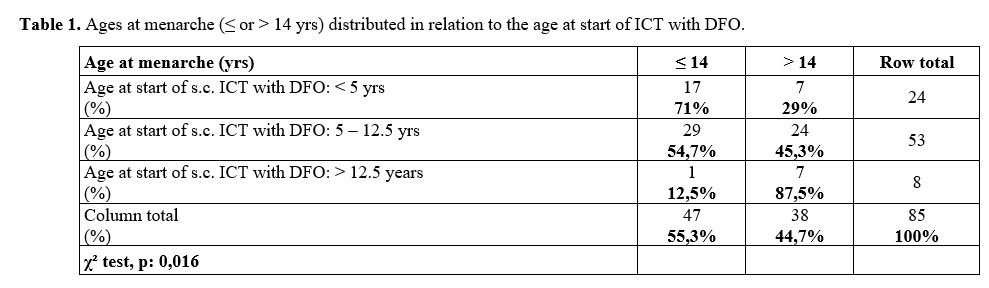 |
Table
1. Ages at menarche (≤ or > 14 yrs) distributed in relation to the age at start of ICT with DFO.
|
At
menarche, the average BMI-Z score of the whole group of patients was
within the normal range (- 0,09 ± 1), whereas, in 47 TDT adolescents
with age at menarche ≤ 14 yrs, it was 0.3 ± 0.85. The difference with
patients who had menarche at age > 14 yrs was statistically
significant (n = 38, - 0,53 ± 1,1; p = 0,001).
Finally, BMI-Z score was inversely correlated with age at start of ICT (n = 67, r = - 039; p = 0,0001) and age at menarche (Figure 2).
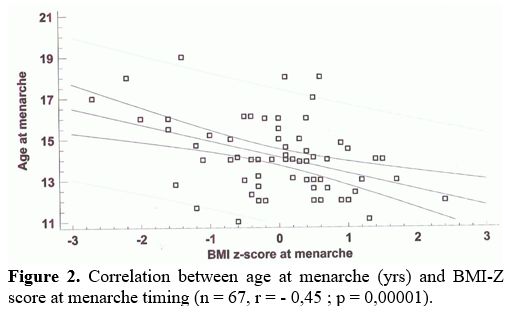 |
Figure 2. Correlation between age at menarche (yrs) and BMI-Z score at menarche timing (n = 67, r = - 0,45 ; p = 0,00001).
|
Menstrual characteristics and duration of menstrual history in relation to BMI and ICT.
The 50 TDT patients with subsequent regular menstrual cycles had a
menarche age lower than 29 patients with irregular menstrual cycles
(13,9 ± 1,8 vs. 14,7 ± 1,9 yrs; p: 0.028). The prevalence of regular
and irregular menstrual cycles, in relation to the age at menarche ≤ or
> 14 years, is reported in table 2.
Age at menarche at or below 14 yrs was associated with a lower risk of
irregular menstrual cycles (38% vs. 62% at age > 14 years, χ² =
0,005).
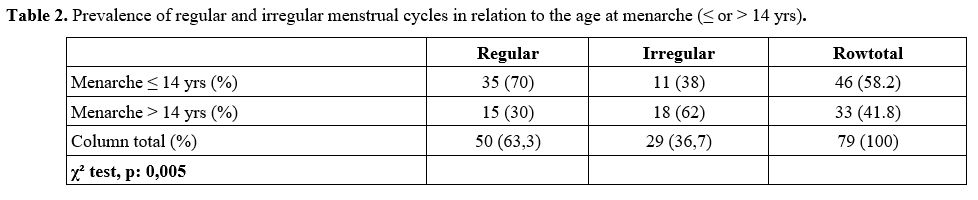 |
Table 2. Prevalence of regular and irregular menstrual cycles in relation to the age at menarche (≤ or > 14 yrs).
|
Nutritional
status of "thinness" at menarche was associated with a lower prevalence
of subsequent regular menstrual cycles (χ² test; p: 0.02). Moreover,
the BMI-Z score at menarche was directly correlated to the number of
years of menstruations (n = 67, r = 0,36, p = 0,003), and the
prevalence of regular menstrual cycles in patients with normal BMI was
higher (68,7%) compared to those with thinness or overweight (38,4%, χ²
test; p: 0,02).
The condition of thinness in 13 TDT patients (2
mild, 7 moderate, and 4 severe) was associated with a higher prevalence
of SA than in the patients with normal BMI (92%, χ² test, p: 0,02).
The
duration of menstrual history in the whole group of patients was
strictly correlated to the age at the start of s.c. ICT with DFO. In
particular it was 12,5 ± 8,9 yrs in 24 patients who started the
chelation therapy < 5 years (13 with preserved menses and 11 with
SA), 7,2 ± 8,8 yrs in 54 patients who started treatment from 5 to 12,5
years, and 3,1 ± 2,3 yrs in 8 patients who started treatment > 12,5
yrs (p= < 0,01).
However, it should be noted that precocious
SA is still present in 11 out of 24 TDT patients who started ICT with
DFO at the early age of 2,8 ± 1,2 yrs.
SA developed at a mean age
of 19,5 ± 5,9 years, when SF was 1,676 ± 996 ng/mL. These values were
not significantly lower compared to patients with preserved menses at
last examination (1,938 ± 837 ng/mL, p = 0,08), but significantly lower
compared to 51 TDT patients with SA who started ICT at later age (3,235
± 2,154 ng/mL; p = 0,03). Finally, their BMI-Z score (0,84 ± 1,27) was
not statistically different compared to BMI-Z score of 8 patients with
preserved menses who started ICT before 5 yrs (0,5 ± 0,8, p = 0,52).
Discussion
Timing
of puberty, menarche, and menstrual patterns are considered indicators
of overall health and self-perception of well-being. They are
influenced by multiple variables such as: genetics, geographic
location, climate, psychological factors, socioeconomic status, body
weight and height, nutrition, body fat, and exercise, as well as the
presence of chronic diseases.[17,18]
In our
patients, the mean age at menarche was associated with an earlier age
at the start of ICT with DFO, lower SF levels, and an average BMI-Z
score, and a higher prevalence of regular subsequent menstrual cycles
and duration of menstrual history.
The significant correlation,
observed in our patients, between age at starting of s.c. ICT and SF at
menarche confirm the importance of early onset of chelation therapy.[19]
Our patients started the iron chelation therapy with DFO at a mean age
of 7,6 ±3,8 yrs and twenty-four of them before the age of 5 (2,8 ± 1,2
yrs). Nevertheless, approximately one-third of patients suffered from
some forms of menstrual irregularities (light or infrequent
menstruation) before developing SA. An absent correlation was observed
between age at SA (mean age of 18,1 ± 3,5 yrs ; range: 12 to 30 yrs)
and severity of overload (SF: 3,163 ± 2,125 ng/mL) (p = 0,51).
Patients
with regular menstrual cycles had a lower mean age at menarche.
Interestingly, age at menarche (≤ 14 yrs) was associated with a lower
risk of irregular menstrual cycles (38% vs. 62% at age > 14 years,
χ² = 0,005). Moreover, lower age at menarche was associated with a
better BMI-Z score, whereas the nutritional status of thinness was
associated with a lower frequency of regular menstrual cycles history
and a higher frequency of SA. However, the SF and BMI-Z score in 11 out
of 62 TDT patients with SA, who started ICT before 5 years of age,
resulted not different compared to patients with preserved menses. The
small number of patients may have influenced the significance between
the two groups, but other factors cannot be excluded.
In all TDT
patients who developed SA, acquired central hypogonadism was diagnosed,
mainly linked to chronic exposure to iron overload. At the SA time,
their mean SF level was 3,163 ± 2,125 ng/mL, whereas, at the last
examination, the SF in patients with preserved menses was 1,938 ± 837
ng/mL (p = 0,03). Hypogonadotropic hypogonadism (HH), also called
central hypogonadism or secondary hypogonadism, is characterized by
deficient Gn-RH secretion from the hypothalamus leading to decreased LH
and FSH secretion from the pituitary gland and inadequate testosterone
(in males) or estradiol (in females) production.
It has been
reported that iron deposition in the pituitary gland starts in the
first decade of life and has a cytotoxic effect, leading to
hypogonadotropic hypogonadism.[20,21]
After
approximately one year of transfusions, iron is deposited in
parenchymal tissues, where it may cause significant toxicity compared
to that within reticuloendothelial cells. Furthermore, as iron loading
progresses, the capacity of serum transferrin, the main transport
protein of iron, to bind and detoxify iron may be exceeded. Therefore,
non–transferrin-bound iron is readily transported through calcium
channels into the liver (hepatocytes), heart (cardiac myocytes), and
endocrine glands, leading to the different clinical complications of
iron overload. In addition, reactive oxygen species produced by the
metabolism of non–transferrin-bound iron contribute to cellular
dysfunction, apoptosis, and necrosis in target organs.[22,23]
Susceptibility
to HH develop¬ment seems to be associated with genotype, as patients
with severe underlying molecular defects have a greater rate of iron
loading and probably a different vulnerability to free radical damage.[24]
Very little is known on the effects of oral ICT on age at menarche,[25]
and nothing is available in the recent literature on the menstrual
history of TDT patients during the treatment with s.c. DFO. Moreover,
the relationship of menstrual history with nutritional status has not
been investigated before.
Despite an early iron chelation
therapy (mean age: 2,8 ± 1,2 yrs), 11 out of 24 of our TDT patients
developed a precocious SA, at a mean age of 19,5 ± 5,9 years with a
mean SF level of 1,758 ± 846 ng/mL. Intensification of treatment or
improvement of patients' compliance to iron chelation therapy did not
induce a reversal of H-P-G dysfunction. In contrast to our
observations, Farmaki et al.[26] reported in four out
of 10 TDT patients with SA an improvement or reversal of
pituitary-gonadal dysfunction after a very intensive combined chelation
with DFO and DFP (the SF in the entire group of patients was 99 ± 37
ng/mL). Two of them became pregnant spontaneously and two after in
vitro fertilization (IVF). However, we do not know how long we can
maintain children and adolescents at normal levels of iron stores
without side effects from the over-chelation of iron.
Interestingly,
we found an inverse correlation between the BMI-Z score and age at
menarche and a direct relationship between the BMI-Z score and years of
menstrual cycles. It is well known that an optimal nutritional status
is important for normal growth and pubertal development.[27]
A
decreased body fat results in a leptin concentration reduction and
gonadotropin deficiency. Leptin is a polypeptide hormone produced in
fat cells due to the expression of the ob gene. In girls, leptin levels
dramatically increase, as puberty develops, and stimulate the H-P-G
axis, and which is a permissive factor in puberty onset.[28]
The
nutritional history of our patients did not document relevant
deficiencies; however, we cannot fully exclude a reduced intake of
macro and micronutrients. Goldberg et al.[29] have
reported that many TDT patients who consumed an adequate dietary intake
had deficient levels of circulating nutrients. Liver iron concentration
in TDT patients displayed a significant negative relationship with
vitamins C, E, and zinc, indicating that in iron-overloaded patients,
these nutrients are either endogenously consumed at higher rates or
sequestered within the liver resulting in a functional nutrient
deficiency. Therefore, even a marginal zinc deficiency could affect
leptin secretion and serum leptin concentrations, as documented in
zinc-deficient rats.[30] A potential additional
factor leading to impaired leptin secretion may be the iron toxicity in
adipose tissue, as reported by Perrone et al.[31]
Although
our paper has some limitations, due to the retrospective nature of the
study, the limited number of patients included in the study, the use of
surrogate indices for assessing iron overload, and the lack of
comparison with other iron chelators, it has the strength of
standardized documentation of clinical data, the rigorous selection of
patients and the description, for the first time, of a long-term
menstrual history in two groups of TDT patients who started early and
late ICT with DFO.
In conclusion, early ICT in TDT patients was
associated with a regular "tempo" of pubertal onset and a higher
frequency of subsequent regular menstrual cycles. However, SA remains a
frequent morbidity and obstacle for having children. Patients with
transfusional iron overload begin to develop pituitary iron overload in
the first decade of life; however, clinically significant volume loss
was not observed until the second decade of life.[20,21]
The serum ferritin levels in our patients with SA were consistent with a non-adequate ICT.
Therefore
optimization of iron burden is mandatory, particularly in subjects
uncompliant to therapy or no access to optimal medical care.
The
present data could represent a reference for future studies in order to
compare also the efficacy of different iron chelation regimes on the
long-term maintenance of a normal function of an H-P-G axis and may
generate future studies for investigating whether body mass percentile
and trace elements could be potential additional factors responsible
for menstrual cycle irregularities in TDT patients.
References
- Weatherall DJ. Thalassemia as a global health
problem: recent progress toward its control in the developing
countries. Ann N Y Acad Sci. 2010; 1202:17-23. https://doi.org/10.1111/j.1749-6632.2010.05546.x PMid:20712767
- Viprakasit
V, Ekwattanakit S. Clinical Classification, Screening and Diagnosis for
Thalassemia. Hematol Oncol Clin North Am. 2018;32:193-211. https://doi.org/10.1016/j.hoc.2017.11.006 PMid:29458726
- Thalassaemia
International Federation. Guidelines for the management of transfusion
dependent thalassaemia (TDT). 3rd ed. 2014 Available from www.thalassaemia.org.cy. Accessed 15 June 2018.
- Kanbour
I, Chandra P, Soliman A, De Sanctis V, Nashwan A, Abusamaan S, Moustafa
A, Yassin MA. Severe Liver Iron Concentrations (LIC) in 24 Patients
with β-Thalassemia Major: Correlations with Serum Ferritin, Liver
Enzymes and Endocrine Complications. Mediterr J Hematol Infect Dis.
2018;10 (1):e2018062. doi: 10.4084/MJHID.2018.062. https://doi.org/10.4084/mjhid.2018.062 PMid:30416694 PMCid:PMC6223579
- Hankins
JS, McCarville MB, Loeffler RB, Smeltzer MP, Onciu M, Hoffer FA, Li CS,
Wang WC, Ware RE, Hillenbrand CM. R2* magnetic resonance imaging of the
liver in patients with iron overload. Blood. 2009;113: 4853-4855. https://doi.org/10.1182/blood-2008-12-191643 PMid:19264677 PMCid:PMC2686136
- St
Pierre TG, El-Beshlawy A, Elalfy M, Al Jefri A, Al Zir K, Daar S, Habr
D, Kriemler-Krahn U, Taher A. Multicenter validation of spin-density
projection-assisted R2-MRI for the non invasive measurement of liver
iron concentration. Magn Reson Med. 2014;71: 2215-2223. https://doi.org/10.1002/mrm.24854 PMid:23821350 PMCid:PMC4238736
- Olivieri NF, Brittenham GM. Iron-chelating therapy and the treatment of thalassaemia. Blood. 1997;89:739-761. https://doi.org/10.1182/blood.V89.3.739
- Berdoukas
V, Farmaki K, Carson S, Wood J, Coates T. Treating thalassemia
major-related iron overload: the role of deferiprone. J Blood Med.
2012;3:119-129. https://doi.org/10.2147/JBM.S27400 PMid:23112580 PMCid:PMC3480237
- Wang
YX, Arvizu M, Rich-Edwards JW, Stuart JJ, Manson JE, Missmer SA, Pan A,
Chavarro JE. Menstrual cycle regularity and length across the
reproductive lifespan and risk of premature mortality: prospective
cohort study. BMJ. 2020; 371: m3464. https://doi.org/10.1136/bmj.m3464 PMid:32998909 PMCid:PMC7526082
- Bronspiegel-Weintrob
N, Olivieri NF, Tyler B, Andrews DF, Freedman MH, Holland FJ. Effect of
age at the start of iron chelation therapy on gonadal function in
β-thalassemia major. N Engl J Med. 1990;323:713-719. https://doi.org/10.1056/NEJM199009133231104 PMid:2388669
- De
Sanctis V, Bernasconi S, Bianchin L, Bona G, Bozzola M, Buzi F, De
Sanctis C, Rigon F, Tatò L, Tonini G, Perissinotto E. Onset of
menstrual cycle and menses features among secondary school girls in
Italy: a questionnaire study on 3783 students. Indian J Endocrinol
Metab. 2014; 18 (suppl 1): S84-S92. https://doi.org/10.4103/2230-8210.140251 PMid:25538883 PMCid:PMC4266874
- Papadimitriou
A, Fytanidis G, Douros K, Bakoula C, Nicolaidou P, Fretzayas A. Age at
menarche in contemporary Greek girls: evidence for levelling-off of the
secular trend. Acta Paediatr 2008; 97:812-815. https://doi.org/10.1111/j.1651-2227.2008.00806.x PMid:18460111
- Rosenfield
RL, Cooke DW, Radovick S. Puberty and its disorders in the female. In:
Sperling MA. Pediatric Endocrinology, fourth edition. ELSEVIER
Saunders, Philadelphia 2014; pp. 694-696. https://doi.org/10.1016/B978-1-4557-4858-7.00024-X
- De
Sanctis V, Soliman AT, Elsedfy H, Skordis N, Kattamis C, Angastiniatis
M, Karimi M, Yassin MA, El Awwa A, Stoeva I, Raiola G, Galati MC,
Bedair EM, Fiscina B, El Kholy M. Growth and endocrine disorders in
thalassemia: the International network on endocrine complications in
thalassemia (I-CET) position statement and guidelines. Indian J
Endocrinol Metab. 2013; 17:8-18. https://doi.org/10.4103/2230-8210.107808 PMid:23776848 PMCid:PMC3659911
- Cole
TJ, Flegal KM, Nicholls D, Jackson AA. Body Mass Index cut offs to
define thinness in children and adolescents: international survey. BMJ.
2007;335(7612):194. https://doi.org/10.1136/bmj.39238.399444.55 PMid:17591624 PMCid:PMC1934447
- Casale
M, Meloni A, Filosa A, Cuccia L, Caruso V, Palazzi G, Gamberini MR,
Pitrolo L, Putti MC, D'Ascola DG, Casini T, Quarta A, Maggio A, Neri
MG, Positano V, Salvatori C, Toia P, Valeri G, Midiri M, Pepe A.
Multiparametric Cardiac Magnetic Resonance Survey in Children With
Thalassemia Major: A Multicenter Study. Circ Cardiovasc Imaging. 2015 ;
8(8):e003230. https://doi.org/10.1161/CIRCIMAGING.115.003230 PMid:26253625
- Wang
Z, Dang S, Xing Y, Li Q, Yan H. Correlation of body mass index levels
with menarche in adolescent girls in Shaanxi, China: a cross sectional
study. BMC Womens Health. 2016;16:61. doi: 10.1186/s12905-016-0340-4. https://doi.org/10.1186/s12905-016-0340-4 PMid:27599475 PMCid:PMC5013571
- Palel L. Growth and Chronic Disease. Ann Nestlé [Engl]. 2007;65:129-136. https://doi.org/10.1159/000112235
- Bronspiegel-Weintrob
N, Olivieri NF, Tyler B, David F, Andrews DF, Melvin H, Freedman MH,
Holland FJ. Effect of age at the start of iron chelation therapy on
gonadal function in β-thalassemia major. N Engl J Med. 1990;
323:713-719. https://doi.org/10.1056/NEJM199009133231104 PMid:2388669
- Wood JC. Guidelines for quantifying iron overload. Hematology (Am Soc Hematol Educ Program). 2014;2014:210-215. https://doi.org/10.1182/asheducation-2014.1.210 PMid:25696857
- Hekmatnia
A, Radmard AR, Rahmani AA, Adibi A, Khademi H. Magnetic resonance
imaging signal reduction may precede volume loss in the pituitary gland
of transfusion-dependent beta-thalassemic patients. Acta Radiol. 2010;
51:71-77. https://doi.org/10.3109/02841850903292743 PMid:20001472
- Pippard M, Johnson D, Callender S, Finch C. Ferrioxamine excretion in iron loaded man. Blood. 1982;60:288-294. https://doi.org/10.1182/blood.V60.2.288.bloodjournal602288 PMid:7093519
- Ruder EH, Hartman TJ, Goldman MB. Impact of oxidative stress on female fertility. Curr Opin Obstet Gynecol. 2009;21:219-222. https://doi.org/10.1097/GCO.0b013e32832924ba PMid:19469044 PMCid:PMC2749720
- Skordis
N, Gourni M, Kanaris C, Toumba M, Kleanthous M, Karatzia N, Pavlides N,
Angastiniotis M. The impact of iron overload and genotype on gonadal
function in women with thalassaemia major. Pediatr Endocrinol Rev.
2004;2:292-295.
- Origa R, Danjou F,
Orecchia V, Zappu A, Dessì C, Foschini ML, Leoni GB, Moi P, Morittu M,
Demurtas A, Loche S. Current growth patterns in children and
adolescents with thalassemia major. Blood. 2016;128: 2580 -2582. https://doi.org/10.1182/blood-2016-05-712695 PMid:27737888
- Farmaki
K, Tzoumari I, Pappa C, Chouliaras G, Berdoukas V. Normalisation of
total body iron load with very intensive combined chelation reverses
cardiac and endocrine complications of thalassaemia major. Br J
Haematol. 2010;148:466-475. https://doi.org/10.1111/j.1365-2141.2009.07970.x PMid:19912219
- P,
Sanguansermsri T, Fuchs GJ. Malnutrition and growth abnormalities in
children with beta thalassemia major. Southeast Asian J Trop Med Public
Health. 1996; 27: 356-361.
- Kiess W,
Reich A, Meyer K, Glasgow A, Deutscher J, Klammt J, Yang Y, Müller G,
Kratzsch J. A role for leptin in sexual maturation and puberty? Horm
Res. 1999; 51 (S3): 55-63. https://doi.org/10.1159/000053163 PMid:10592445
- Goldberg
EK, Neogi S, Lal A, Higa A, Fung E. Nutritional deficiencies are common
in patients with Transfusion-Dependent Thalassemia and associated with
iron overload. J Food Nutr Res (Newark). 2018;6: 674-681. https://doi.org/10.12691/jfnr-6-10-9 PMid:30569002 PMCid:PMC6296481
- Ott
ES, Shay NF. Zinc deficiency reduces leptin gene expression and leptin
secretion in rat adipocytes. ExpBiol Med (Maywood). 2001;226:841-846. https://doi.org/10.1177/153537020122600906 PMid:11568307
- Perrone
L, Perrotta S, Raimondo P, Mucerino J, De Rosa C, Siciliani MC, Santoro
N, Miraglia del Giudice E. Inappropriate leptin secretion in
thalassemia: a potential cofactor of pubertal timing derangement. J
Pediatr Endocrinol Metab. 2002; 16:877-881. https://doi.org/10.1515/JPEM.2003.16.6.877 PMid:12948300
[TOP]