Matteo G. Della Porta1 and Cristina Picone2
1 Cancer Center, Humanitas Research Hospital & Humanitas University, Rozzano – Milan, Italy
2 Department of Hematology Oncology, Fondazione IRCCS Policlinico San Matteo, Pavia Italy
Corresponding
author: Prof. Matteo Giovanni Della Porta, MD, Cancer Center,
Humanitas Research Hospital & Humanitas University, via Manzoni, 56
Rozzano – Milan, Italy. Tel: +39 0282247668, Fax: +39 0282244590.
E-mail:
matteo.della_porta@hunimed.eu
Published: 15 February, 2017
Received: November 14, 2016
Accepted: January 20, 2017
Mediterr J Hematol Infect Dis 2017, 9(1): e2017017 DOI
10.4084/MJHID.2017.017
This article is available on PDF format at:
This is an Open Access article distributed
under the terms of the Creative Commons Attribution License
(https://creativecommons.org/licenses/by-nc/4.0),
which permits unrestricted use, distribution, and reproduction in any
medium, provided the original work is properly cited.
|
Abstract
The
pathological hallmark of myelodysplastic syndromes (MDS) is marrow
dysplasia, which represents the basis of the WHO classification of these
disorders. This classification provides clinicians with a useful tool
for defining the different subtypes of MDS and individual prognosis. The
WHO proposal has raised some concern regarding minimal diagnostic
criteria particularly in patients with normal karyotype without robust
morphological markers of dysplasia (such as ring sideroblasts or excess
of blasts). Therefore, there is clearly need to refine the accuracy to
detect marrow dysplasia. Flow cytometry (FCM) immunophenotyping has
been proposed as a tool to improve the evaluation of marrow dysplasia.
The rationale for the application of FCM in the diagnostic work up of
MDS is that immunophenotyping is an accurate method for quantitative
and qualitative evaluation of hematopoietic cells and that MDS have
been found to have abnormal expression of several cellular antigens. To
become applicable in clinical practice, FCM analysis should be based on
parameters with sufficient specificity and sensitivity, data should be
reproducible between different operators, and the results should be
easily understood by clinicians. In this review, we discuss the most
relevant progresses in detection of marrow dysplasia by FCM in MDS
|
The Diagnosis of Myelodysplastic Syndromes
Myelodysplastic
syndromes (MDS) are a group of disorders clinically characterized by
peripheral cytopenia, followed by a progressive impairment in the
ability of myelodysplastic stem cells to differentiate and an
increasing risk of evolution into acute leukemia.[1]
MDS
represent one of the most common hematologic malignancies in Western
countries. They typically occur in elderly people with a median age at
diagnosis of 70 to 75 years in most series, and their annual incidence
exceeds 20 per 100,000 persons over the age of 70 years.[1]
The clinical course of the disease is very heterogeneous, ranging from
indolent conditions spanning years to forms rapidly progressing to
leukemia.[2] This heterogeneity reflects the complexity of the underlying genetic defects.[3]
According
to the prevailing dogma, clonal transformation in MDS would occur at
the level of a committed myeloid stem cell that can give rise to red
cells, platelets, granulocytes and monocytes.[4] The
biologic hallmark of these stem cells is, rather, dysplasia, which
indicates a defective capacity for self-renewal and differentiation and
relies on various morphological abnormalities. Karyotypic aberrancies
(involving loss of genetic material and less frequently balanced
translocations) are detected in about 50% of primary MDS, and when
present are a marker of clonal hematopoiesis.[5]
Important steps have recently been made in characterizing the molecular basis of MDS.[3]
MDS del(5q) appears to derive from haplo-insufficiency of genes mapping
to chromosome 5q32- q33, in particular from reduced expression of RPS14
and miR-145/-146a, and from mutations of Casein Kinase 1A1 and TP53
genes.[6] In addition, acquired somatic mutations have
been detected in several genes, including TET2, ASXL1, CBL, ETV6, EZH2,
IDH1, IDH2, KRAS, NPM1, NRAS, RUNX1, and TP53.[4] More
recently, genes encoding for spliceosome components were identified in
a high proportion of patients with MDS. These genes include SF3B1,
SRSF2, U2AF35 and ZRSR2, and to a lesser extent, SF3A1, SF1, U2AF65 and
PRPF40B.[7]
Although most of the mutated genes in
MDS can be detected in different myeloid neoplasms and are not specific
for MDS, they may be of value to provide evidence for a clonal disorder
in patients with suspected MDS. In a recent comprehensive report,[7]
a total of 52% of patients with normal cytogenetics had at least one
point mutation. These figures are even higher when accounting for
mutations of the genes encoding for splicing factors. Although the
spread of massive genotyping methods will soon make possible for
clinicians to detect a broad range of in peripheral blood at a
reasonable cost, the screening of such molecular defects cannot be
recommended at this stage on a routine basis.[7]
To
date, the morphological evaluation of marrow dysplasia represents the
basis of the World Health Organization (WHO) classification of these
disorders.[8] This classification provides clinicians
with a very useful tool for defining the different subtypes of MDS and
determining individual prognosis. The combination of overt marrow
dysplasia and clonal cytogenetic abnormalities allows a conclusive
diagnosis of MDS. However, this combination is found only in some
patients, who tend to be those with more advanced disease. In many
instances, cytogenetics is not informative so that the diagnosis of MDS
is based entirely and exclusively on morphological evaluation.[8]
The WHO proposal has raised some concern regarding minimal diagnostic criteria for formulating the diagnosis of MDS.[9]
Morphology may be difficult to evaluate, because cellular abnormalities
of bone marrow cells are not specific for MDS and may be found in other
pathological conditions.[10,11] As a consequence, in
clinical practice inter-observer reproducibility for recognition of
dysplasia is usually poor, particularly in patients who do not have
robust morphological markers such as ring sideroblasts or excess of
blasts.[11] Moreover, poor technical quality of the
specimen is a common obstacle in the accurate morphological diagnosis
of MDS and also has an influence on the diagnostic yield of
conventional cytogenetics. Finally, morphology may be difficult to
evaluate in some patients either due to hypocellularity or fibrosis of
the marrow.[12]
Rationale for the Application of Flow Cytometry in the Diagnostic Work-Up of Patients with Suspected MDS
Flow
cytometry (FCM) immunophenotyping was introduced by WHO proposal for
the classification of hematologic neoplasms as an indispensable tool
for the diagnosis, classification, staging, and monitoring of several
diseases, such as lymphoproliferative disorders and acute leukemias.[13]
In addition, immunophenotyping has been proposed in last years as a
tool to improve the evaluation of marrow dysplasia. Rationale for the
application of FCM in the diagnostic work up of MDS is that: i)
immunophenotyping is an accurate method for quantitative and
qualitative evaluation of hematopoietic cells (in this context it
should be underlined that however, the morphologic definition of bone
marrow cells is not equal to and cannot be used in an exchangeable
manner with flow cytometric nomenclature) and, ii) MDS have been found
to have abnormal expression of several cellular antigens.[13-15]
Flow cytometry immunophenotyping is able to identify specific
aberrations in both the immature and mature compartments among
different bone marrow hematopoietic cell lineages.[16-20]
Although no single immunophenotypic parameter has been proven to be
diagnostic of MDS, combinations of such parameters into scoring systems
have been shown to discriminate MDSs from other cytopenias with high
sensitivity and acceptable specificity.
Flow cytometry was proven
to be highly sensitive in identifying patients likely to be suffering
from a clonal disease process (ie, an MDS lacking specific diagnostic
markers such as excess blasts, ring sideroblasts or karyotypic
aberrations) rather than cytopenia of undetermined significance, which
includes cases of sustained cytopenias in one or more lineages that do
not meet the minimal criteria for MDS and cannot be explained by any
other hematologic or nonhematologic disease.[16-20] In
addition, flow cytometry is useful for distinguishing refractory anemia
from refractory cytopenia with multilineage dysplasia by identifying
immunophenotypic abnormalities in myeloid and monocytic compartments.[16-20]
Although
further prospective validation of markers and immunophenotypic patterns
against control patients with secondary dysplasia and further
standardization in multicenter studies are required, at present, flow
cytometry abnormalities involving one or more of the myeloid lineages
can be considered as suggestive of MDS.
Standard methods for cell
sampling, handling, and processing, and minimal combinations of
antibodies for flow cytometry analysis of dysplasia in MDS have
recently been established by the International Flow Cytometry Working
Group within the European LeukemiaNet.[21]
The
integration of flow cytometry immunophenotyping following these
standards is recommended in the workup of patients with suspected MDS
by the European LeukemiaNet guidelines for diagnosis and treatment of
primary MDS,[22] although the implementation of these procedures may not be immediately feasible in some hematologic centers.
In this report, we reviewed the most relevant advancements in the evaluation of marrow dysplasia by FCM in MDS.
Immunophenotypic Evaluation of Myeloid Dysplasia
Morphological
granulocytic dysplasia as defined by WHO criteria is present in about
60% of MDS patients at diagnosis.[8,9] Most significant morphological
alterations on granulocytic lineage included hypogranularity on myeloid
cells, the presence of pseudo-pelger neutrophils and increased
prevalence in bone marrow of myeloid cells in the earliest stage of
maturation.[11] These abnormalities significantly
affected the detection of physical parameters (i.e., side scatter, SSC
and forward scatter, FSC) by FCM.[23] Defective
capacity for self-renewal and differentiation by myelodysplastic stem
cells also relies on various abnormalities of antigen expression on
granulocytic cells, which may be easily detected by FCM due to a large
availability of specific antibodies for myeloid lineage.[16-19]
Reported aberrancies of granulocytic lineage include the presence of
antigens that are not normally present, such as lymphoid antigens, and
altered expression of myeloid antigens, either in a single population
of cells or within a generation of maturing cells. Furthermore,
monocytic compartment is also affected in MDS.[16-19]
Davis
studied for the first time the pattern of CD16 and CD11b expression by
maturing granulocytes in the bone marrow of patients with MDS and
healthy controls.[15] There was a highly consistent
normal pattern of CD11b and CD16 expression in the granulocytic series
in healthy subjects, while in MDS patients an increased percentage of
granulocytic cells with low CD16 or both low CD16 and low CD11b was
noticed.[15] In addition, an altered granulocytic maturation pattern can be demonstrated by plotting CD13 versus CD16.[16-19]
During maturation; myeloid cells normally acquire increasing levels of
CD16 that are initially accompanied by a decrease in CD13 expression as
cells mature from blasts through the myelocyte and metamyelocyte stages
of maturation, followed by intermediate levels of CD13 in band forms
and high levels in segmented neutrophils. Several abnormalities on
CD13/CD16 maturation pattern were described in MDS patients, including
an increase of cells in myelocyte and metamyelocyte stages of
maturation and a decrease of CD13+CD16+ neutrophils.[16-19]
Although
these investigations defined immunophenotypic abnormalities in MDS,
they did not address the potential contribution of FCM to the diagnosis
of MDS. The study of Stetler-Stevenson et al. published in 2001 was the
first to demonstrate that the identification of immunophenotypic
abnormalities by FCM is useful in establishing a diagnosis of a MDS,
especially when the results of the morphologic evaluation and
cytogenetic studies are indeterminate.[16] In
addition to maturation abnormalities, aberrancies in the expression of
several antigens on granulocytes such as CD64, CD10, and CD56 were
described in MDS. Lymphoid antigens, such as CD2, CD5, CD7, and CD19
may be abnormally expressed on myeloid progenitors and maturing myeloid
cells. Moreover, a common finding in these patients is the atypical
expression of antigens on immature myeloid cells that are normally
expressed on mature myeloid cells, such as CD11b and/or CD15.[17-19]
As
far as monocytic compartment is concerned, most frequent abnormalities
observed in MDS patients include altered expression of CD56, HLA-DR,
CD36, CD33, CD15, CD14, CD13, and CD11b.[18,19,24]
In general, the amount of abnormalities reported by FCM correlates with
the degree of dysplasia assessed by morphology. Although most of the
studies have evaluated bone marrow cells, there is some evidence that
FCM analysis of peripheral blood could also assist in the diagnosis of
MDS.[25] Scientific evidence suggests that aberrant
antigen expression by myeloid cells is more frequent and carries more
discriminant weight on detection of marrow dysplasia than altered
expression of monocytic antigens.[24] A single myeloid immunophenotypic abnormality was reported in about 30–40% of patients affected with nonclonal cytopenia.[16-20]
Therefore, a single myeloid immunophenotypic abnormality is not a
definitive finding for MDS, and other abnormalities should be detected
on granulocytic cells to conclude that myeloid dysplasia is present.
Multiparametric evaluation of myeloid and monocytic maturation and
antigen expression pattern leads to the identification of two or more
aberrancies in the great majority of MDS cases (from 70% to more than
90% in different studies).[16-20,26]
In general FCM is more sensitive in detection of myeloid dysplasia with
respect to morphology, and immunophenotypic myeloid abnormalities are
identified in a significant percentage of cases (from 20% to more than
90%) classified as refractory cytopenia with unilineage dysplasia or
unclassifiable MDS.[16-20,26] In
addition, FCM was found to be useful for detection of marrow dysplasia
in a proportion of patients with marrow hypocellularity, fibrosis or
inadequate specimen collection, suggesting that variables related to
sample quality are less significant in immunophenotypic analysis than
in morphological evaluation.[17]
The great
variability on the percentage of reported immunophenotypic
abnormalities in MDS patients reflect in part the biological
heterogeneity within these disorders, but more likely, the lack of a
standardized and reproducible procedure for the evaluation of these
parameters.[21] The most largely used approach to evaluate myeloid dysplasia by FCM is pattern recognition analysis.[16]
This is a qualitative method based on recognition of a deviation from
normal antigen expression pattern. Although similarly to morphological
evaluation this approach is a good tool for expert operators (i.e.,
people with extensive knowledge of changes in antigen expression in
normal and pathological hematopoietic cell differentiation) pattern
recognition analysis presents several weak points. The numerical
description of the results is difficult, thus quantitative analysis is
not possible; moreover, the precise definition of the normal pattern of
reference may be complex.[13] Overall, FCM
multiparametric approaches based on a quantitative evaluation of
myeloid antigens allow to classify about 90% correctly of cases with
suspected MDS.[16-20,26] The ELN
working group for FCM in MDS started a consensus process on how to
standardize sample collection/ preparation and data acquisition, that
is expected to significantly improve the FCM accuracy in detection of
marrow dysplasia.[21,27-30] Immunophenotypic Analysis of Blast Cells
Clonal
transformation in MDS occurs at the level of a myeloid committed stem
cell which has a competitive advantage over normal stem cell
compartment.[1] These hematopoietic precursors
(blasts) are morphologically defined as ‘‘immature cells with
uncondensed chromatin pattern, prominent nucleoli, low
nuclear/cytoplasmic ratio, and no/few cytoplasmic granules’’.[11]
The evaluation of blast compartment has diagnostic relevance in the WHO
system, and the percentage of marrow blasts has recognized to have
prognostic effect by all the currently available prognostic scores.[8]
In the WHO guidelines, despite inaccuracies inherent in manual
differential counting, morphological analysis is actually the gold
standard for determining blast percentage.[11] The
first attempt of FCM immunophenotyping was to provide a quantitative
estimation of bone marrow blasts with increased sensitivity and
reproducibility with respect to morphological count. Unfortunately, the
quantitative evaluation of marrow blasts in MDS by FCM presents both
technical and intrinsic limitations.[13] First, MDS
blasts are not predominant cells in the bone marrow making their
reliable analysis difficult, and in addition they are identified in the
CD45 versus SSC dotplot as CD45lowSSClow cells; however, hypogranular
more mature myeloid cells may have decreased SSC and fall in this
region, and it may be difficult to distinguish monoblasts from more
mature monocytes.[13] The percentage of CD34+ cells
determined by FCM has been tested as a substitution for a visual blast
count. However, although hematopoietic cells that express CD34 are
blasts, not all blasts express CD34. It should be considered in
addition that marrow samples for morphological evaluation can differ
form that for FCM analysis in terms of cellularity . Hence, the percent
of CD34+ cells determined by FCM as substitution for a visual blast
count in MDS is discouraged by current WHO classification.[8,31]
More
interesting results in the light of a diagnostic application of FCM in
work-up of MDS patients derive from the analysis of immunophenotypic
abnormalities of blast cell compartment. The proportion of CD34+ cells
is significantly higher in MDS with respect to healthy subjects, and
the great majority of cells are committed to the myeloid lineage
(CD38+HLA-DR+CD13+CD33+).[14,32] In
addition, a significant down-regulation of B-cell lineage-affiliated
genes was observed in CD34+ hematopoietic precursors isolated from
low-risk MDS with respect to healthy controls and patients with
nonclonal cytopenia, and a reduction in stage I hematogones is one of
most consistent immunophenotypic findings in MDS patients.[33,34]
In different studies considering patients performing bone marrow
evaluation for peripheral blood cytopenia, a significant decrease of
CD34+ B cell progenitors was observed in 40–70% of subjects with a
conclusive diagnosis of MDS and in 20–40% of patients with nonclonal
cytopenia. The analysis of both percentages of CD34+ myeloblasts and
CD34+ B cell precursors was found to have little interobserver
variability.[33,34]
Several other
immunophenotypic abnormalities on MDS blast cells were reported,
including asynchronous co-expression of stem-cell and late-stage
myeloid antigens (CD117, CD15, and CD11b) or abnormal expression of
lymphoid markers (CD2, CD5, CD7, CD19, and CD56).[18,19,32,33,35]
However, most of these parameters do not have adequate reproducibility
in the MDS setting with the exception of lymphocytes- to-myeloblasts
CD45 ratio that ensures acceptable interobserver variability by
adjusting data on target cells with those on lymphocytes in the same
sample.
The analysis of percentage of CD34+ myeloblasts, CD34+
B-cell progenitors and myeloblast CD45 expression by FCM has little
interoperator variability and appears to be applicable in many
laboratories.[36,37] When combined together with the
evaluation of SSC on granulocytes, these parameters differentiate
correctly the majority of MDS and pathological controls, sensitivity
ranging from 30 to 70% and specificity ranging from 80% to more than
90% in different studies.[36-38] (Figure 1)
All these findings strongly suggest that CD34-related parameters are
good candidates for the identification of diagnostic markers that not
only can be used for the diagnosis of MDS patients but also are
relatively stable and result in acceptable between-operator data
variation.
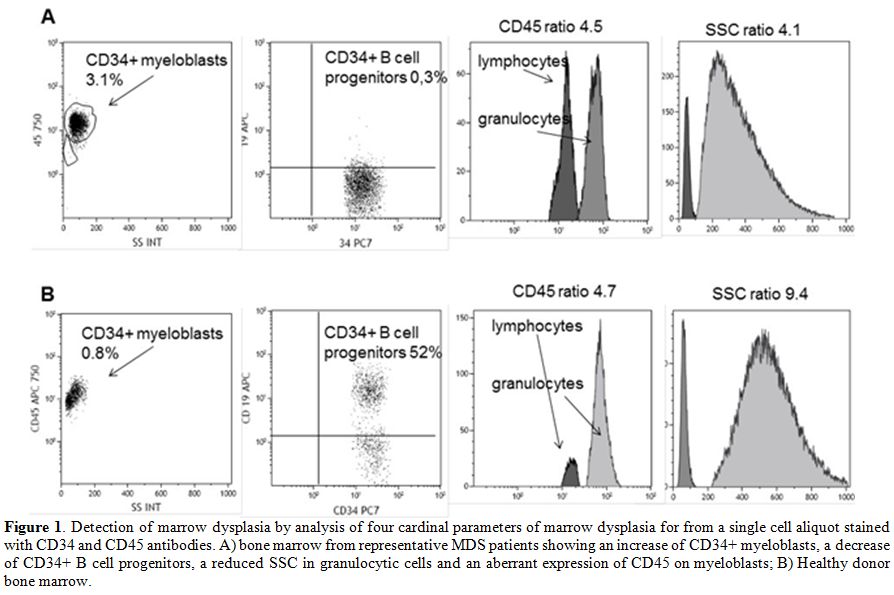 |
Figure 1. Detection of marrow dysplasia by
analysis of four cardinal parameters of marrow dysplasia for from a
single cell aliquot stained with CD34 and CD45 antibodies. A) bone
marrow from representative MDS patients showing an increase of CD34+
myeloblasts, a decrease of CD34+ B cell progenitors, a reduced SSC in
granulocytic cells and an aberrant expression of CD45 on myeloblasts;
B) Healthy donor bone marrow. |
Immunophenotypic Evaluation of Erythroid Dysplasia
Erythroid
dysplasia is the milestone of the morphological diagnosis of MDS. In
fact, it is present in almost all patients with MDS and is the only
morphological abnormality in those with refractory or sideroblastic
anemia. [8,11]
The evaluation
of erythroid dysplasia represents a challenge in the immunophenotypic
analysis of myelodysplastic marrows: the precise identification of
marrow erythroid precursors is problematic, and there is a limited
availability of specific markers.[16]
The first
critical issue of erythroid compartment immunophenotyping is the gating
strategy to identify marrow erythroid precursors.[21,27,39]
Nucleated erythroid cells are characterized by reduced/absent CD45 and
low SSC. To gate CD45dim to negative/SSClow cells is certainly simple
and seems likely to be reproducible. However, this region also contains
mature (anucleate) red cells, cellular debris, and nonhematopoietic
cells, which are not discriminable on the basis of CD45 or scatter
proprieties. Alternatively, an immunological gate based on the antigens
expressed by erythroid cells can be performed. During physiological
development from the basophilic erythroblast to the erythrocyte, there
is a progressive decrease in CD45 expression.[21,27,39]
An increase in glycophorin A (Gly A) is observed early upon
differentiation from the basophilic erythroblast to the orthochromic
erythroblast. Finally, CD71 is one of the earlier antigens expressed
during erythroid maturation (which anticipates Gly A expression),
remains on the reticulocyte after enucleation and then is lost prior to
the loss of the RNA. From a theoretical point of view, gating
erythroblast on the basis of CD71 expression would be preferable, Gly
Aþ cells excluding a proportion of more immature erythroid precursors,
which may be increased in MDS.[21,27,39]
However, a dysregulation of CD71 expression is reported in MDS, and Gly
A that has a very tight coefficient of variation of intensity from
individual to individual should be preferentially adopted in gating
erythroid precursors in the setting of MDS. The lysis process is also
critical, affecting nucleated as well as mature red blood cells to an
unknown variable degree.[27,30,39]
Although a no-lyse, no-wash system would provide the most accurate
estimate of the nucleated red cell, a lyse no-wash approach is
certainly simpler and more easily implementable in the diagnostic
workup of MDS patients. [21,27,39]
The
study by Stetler-Stevenson et al. demonstrated for the first time the
feasibility of the evaluation of erythroid dysplasia by FCM.[16]
However, the only consistent erythroid abnormality in this study was a
dys-synchronous expression of CD71 versus Gly A on red cell precursors.
In
last years an increasing amount of studies addressed the issue of the
immunophenotypic evaluation of erythroid compartment in MDS.[40-43]
Flow cytometric aberrancies that have been reported to reflect
MDS-related dyserythropoiesis are: a) an increased number of nucleated
erythroid cells within total nucleated cells; b) an altered proportion
of consecutive erythroid differentiation stages, such as an increased
number of immature erythroid cells (CD117+ and/or CD105+) or, by
contrast, a decrease in erythroid progenitors; c) an abnormal pattern
of CD71 versus CD235a; d) a reduced expression of CD71 and/or CD36; and
e) an overexpression of CD105. Most of these aberrancies are present in
70–80% of MDS cases.[40-43] The ELN working group for
FCM in MDS recently reported the results of a multicenter study focused
on defining those erythroid FCM parameters that enable distinction of
dyserythropoiesis associated with MDS from non-clonal cytopenias.[43]
Analysis of the presence of aberrancies in the erythroid markers CD71
and CD36 (expressed as the coefficient of variation, CV), together with
the MFI of CD71 and an abnormal percentage of CD117+ erythroid
progenitor cells provided the best discrimination between MDS and
non-clonal cytopenia. A weighted score based on these four parameters
yielded a specificity of 90% and a sensitivity of 33%.
Addition of
erythroid aberrancies to flow cytometric models based on the evaluation
of myeloid abnormalities may significantly increase the sensitivity to
detect myelodysplastic changes in bone marrow.[40-43]
Conclusions
The
implementation of WHO classification of MDS in clinical practice
compels a refinement of the accuracy to detect marrow dysplasia.[8] FCM immunophenotyping has been proposed as a tool to improve the evaluation of marrow dysplasia.[13] To become clinically applicable,FCM
analysis should be based on parameters with sufficient specificity and
sensitivity, data should be reproducible between different operators,
and the results should be easily understood by clinicians.[13,15]
With respect to this ideal situation, the results of the studies that
pointed out the feasibility of immunophenotyping in diagnostic work-up
of MDS patients raise some concerns: no single marker has proved able
to discriminate accurately between MDS and other pathological
conditions, no consensus exists on which diagnostic parameters are the
most appropriate, and published protocols are mainly based on a
qualitative analysis of cytometric variables thus limiting a wide
clinical implementation.[21,27,29]However,
in recent years significant progresses were made. Clonal transformation
in MDS occurs at the level of a CD34+ committed stem cell, and
therefore CD34-related parameters are good candidates for
identification of diagnostic markers for these disorders.[4,31,32]
Consistent immunophenotypic aberrations reported in MDS CD34+ cell
compartment are an increase of CD34+ myeloblasts, a decrease of B cell
progenitors, expression of lymphoid antigens and abnormal CD45
expression. Increasing evidence suggests that these parameters have
little interoperator variability and, when combined, are able in
discriminating between MDS and patients with nonclonal cytopenia.[31,37]
Evaluation
of erythroid dysplasia represents a challenge in the immunophenotypic
analysis of myelodysplastic marrows due to a limited availability of
specific markers.[16] Promising results are coming
from recent studies, showing that the addition of erythroid aberrancies
to flow cytometric models based on the evaluation of myeloid
abnormalities may significantly increase the sensitivity to detect
myelodysplastic changes in bone marrow.[40-43]A
standardized application of FCM in the diagnosis of MDS also requires a
minimal variability in sample processing, antibody combinations, and
data acquisition. The European LeukemiaNET (ELN) working group for FCM
in MDS started a consensus process on how to standardize sample
collection/preparation and data acquisition. It is expected to
significantly improve the diagnostic accuracy of FCM in MDS.[21,27,28,29]According
to the available evidence and published diagnostic guidelines, in
clinical practice immunophenotyping is strongly indicated in the
screening evaluation of patients with peripheral blood cytopenia:[13,22]
in this clinical situations, it can provide a sensitive screen for the
presence of hematologic malignancy and/or assist in demonstrating the
absence of disease. In addition, when morphology and cytogenetics are
indeterminate, an abnormal phenotype determined by FCM can help to
establish a definitive diagnosis of MDS.[13,22] References
- Rollison DE, Howlader N, Smith MT, et al.
Epidemiology of myelodysplastic syndromes and chronic
myeloproliferative disorders in the United States, 2001-2004, using
data from the NAACCR and SEER programs. Blood. 2008;112:45-55. https://doi.org/10.1182/blood-2008-01-134858 PMid:18443215
- Cazzola
M, Della Porta MG, Travaglino E, et al. Classification and prognostic
evaluation of myelodysplastic syndromes. Semin Oncol. 2011;38:627-634 https://doi.org/10.1053/j.seminoncol.2011.04.007 PMid:21943669
- Cazzola
M, Della Porta MG, Malcovati L. The genetic basis of myelodysplasia and
its clinical relevance. Blood. 2013;122:4021-4023 https://doi.org/10.1182/blood-2013-09-381665 PMid:24136165 PMCid:PMC3862275
- Bejar
R, Levine R, Ebert BL. Unraveling the molecular pathophysiology of
myelodysplastic syndromes. J Clin Oncol 2011;29:504-515. https://doi.org/10.1200/JCO.2010.31.1175 PMid:21220588 PMCid:PMC3969457
- Malcovati
L, Della Porta MG, Pascutto C, et al. Prognostic factors and life
expectancy in myelodysplastic syndromes classified according to WHO
criteria: A basis for clinical decision making. J Clin Oncol
2005;23:7594-7603. https://doi.org/10.1200/JCO.2005.01.7038 PMid:16186598
- Ebert
BL, Pretz J, Bosco J, Chang CY, Tamayo P, Galili N, Raza A, Root DE,
Attar E, Ellis SR, and others. Identification of RPS14 as a 5q-
syndrome gene by RNA interference screen. Nature 2008;451:335-339. https://doi.org/10.1038/nature06494 PMid:18202658 PMCid:PMC3771855
- Papaemmanuil
E, Gerstung M, Malcovati L et al. Clinical and biological implications
of driver mutations in myelodysplastic syndromes. Chronic Myeloid
Disorders Working Group of the International Cancer Genome Consortium.
Blood. 2013;122:3616-3627. https://doi.org/10.1182/blood-2013-08-518886 PMid:24030381 PMCid:PMC3837510
- Arber
DA, Orazi A, Hasserjian R. et al. The 2016 revision to the World Health
Organization classification of myeloid neoplasms and acute leukemia.
Blood. 2016;127:2391- 2405. https://doi.org/10.1182/blood-2016-03-643544 PMid:27069254
- Ramos
F, Fernandez-Ferrero S, Suarez D, et al. Myelodysplastic syndrome: A
search for minimal diagnostic criteria. Leuk Res 1999;23:283-290. https://doi.org/10.1016/S0145-2126(98)00166-0
- Mufti
GJ, Bennett JM, Goasguen J, et al. Diagnosis and classification of
myelodysplastic syndrome: International Working Group on Morphology of
myelodysplastic syndrome (IWGM-MDS) consensus proposals for the
definition and enumeration of myeloblasts and ring sideroblasts.
Haematologica 2008;93: 1712-1717. https://doi.org/10.3324/haematol.13405 PMid:18838480
- Della
Porta MG, Travaglino E, Boveri E, et al. Minimal morphological criteria
for defining bone marrow dysplasia: a basis for clinical implementation
of WHO classification of myelodysplastic syndromes. Leukemia.
2015;29:66-75. https://doi.org/10.1038/leu.2014.161 PMid:24935723
- Della
Porta MG, Malcovati L, Boveri E, et al. Clinical relevance of bone
marrow fibrosis and CD34-positive cell clusters in primary
myelodysplastic syndromes. J Clin Oncol 2009;27:754-762. https://doi.org/10.1200/JCO.2008.18.2246 PMid:19103730
- Craig FE, Foon KA. Flow cytometric immunophenotyping for hematologic neoplasms. Blood 2008;111:3941-3967. https://doi.org/10.1182/blood-2007-11-120535 PMid:18198345
- Ogata
K, Nakamura K, Yokose N, et al. Clinical significance of phenotypic
features of blasts in patients with myelodysplastic syndrome. Blood
2002;100:3887-3896. https://doi.org/10.1182/blood-2002-01-0222 PMid:12393641
- Davis
BH, Foucar K, Szczarkowski W, et al. U.S.-Canadian Consensus
recommendations on the immunophenotypic analysis of hematologic
neoplasia by flow cytometry: Medical indications. Cytometry
1997;30:249-263 https://doi.org/10.1002/(SICI)1097-0320(19971015)30:5<249::AID-CYTO6>3.0.CO;2-C
- Stetler-Stevenson
M, Arthur DC, Jabbour N, et al. Diagnostic utility of flow cytometric
immunophenotyping in myelodysplastic syndrome. Blood 2001;98:979-987. https://doi.org/10.1182/blood.V98.4.979 PMid:11493442
- Malcovati
L, Della Porta MG, Lunghi M, et al. Flow cytometry evaluation of
erythroid and myeloid dysplasia in patients with myelodysplastic
syndrome. Leukemia 2005;19:776- 783. https://doi.org/10.1038/sj.leu.2403680 PMid:15789068
- Wells
DA, Benesch M, Loken MR, et al. Myeloid and monocytic dyspoiesis as
determined by flow cytometric scoring in myelodysplastic syndrome
correlates with the IPSS and with outcome after hematopoietic stem cell
transplantation. Blood 2003;102:394-403. https://doi.org/10.1182/blood-2002-09-2768 PMid:12649150
- van
de Loosdrecht AA, Westers TM, Westra AH, Drager AM, van der Velden VH,
Ossenkoppele GJ. Identification of distinct prognostic subgroups in
low- and intermediate-1- risk myelodysplastic syndromes by flow
cytometry. Blood 2008;111:1067-1077. https://doi.org/10.1182/blood-2007-07-098764 PMid:17971483
- Kern
W, Haferlach C, Schnittger S, et al. Clinical utility of multiparameter
flow cytometry in the diagnosis of 1013 patients with suspected
myelodysplastic syndrome: Correlation to cytomorphology, cytogenetics,
and clinical data. Cancer 2010;116:4549-45633 https://doi.org/10.1002/cncr.25353 PMid:20572043
- van
de Loosdrecht AA, Alhan C, Bene MC, et al. Standardization of flow
cytometry in myelodysplastic syndromes: Report from the first European
LeukemiaNet working conference on flow cytometry in myelodysplastic
syndromes. Haematologica 2009;94:1124- 1134. https://doi.org/10.3324/haematol.2009.005801 PMid:19546437 PMCid:PMC2719035
- Malcovati
L, Hellström-Lindberg E, Bowen D, et al. Diagnosis and treatment of
primary myelodysplastic syndromes in adults: recommendations from the
European LeukemiaNet. Blood. 2013;122:2943-2964 https://doi.org/10.1182/blood-2013-03-492884 PMid:23980065 PMCid:PMC3811170
- Satoh
C, Dan K, Yamashita T, et al. Flow cytometric parameters with little
interexaminer variability for diagnosing low-grade myelodysplastic
syndromes. Leuk Res 2008;32:699-707. https://doi.org/10.1016/j.leukres.2007.08.022 PMid:17936901
- Stachurski
D, Smith BR, Pozdnyakova O, et al. Flow cytometric analysis of
myelomonocytic cells by a pattern recognition approach is sensitive and
specific in diagnosing myelodysplastic syndrome and related marrow
diseases: Emphasis on a global evaluation and recognition of diagnostic
pitfalls. Leuk Res 2008;32:215-224. https://doi.org/10.1016/j.leukres.2007.06.012 PMid:17675229
- Vikentiou
M, Psarra K, Kapsimali V, et al. Distinct neutrophil subpopulations
phenotype by flow cytometry in myelodysplastic syndromes. Leuk Lymphoma
2009;50:401-409. https://doi.org/10.1080/10428190902755497 PMid:19294559
- Alhan
C, Westers TM, Cremers EM, et al. The myelodysplastic syndromes flow
cytometric score: a three-parameter prognostic flow cytometric scoring
system. Leukemia. 2016;30:658-665. https://doi.org/10.1038/leu.2015.295 PMid:26503643
- Westers
TM, Ireland R, Kern W, et al. Standardization of flow cytometry in
myelodysplastic syndromes: a report from an international consortium
and the European LeukemiaNet Working Group. Leukemia.
2012;26:1730-1741. https://doi.org/10.1038/leu.2012.30 PMid:22307178
- Lacombe
F, Bernal E, Bloxham D, et al. Harmonemia: a universal strategy for
flow cytometry immunophenotyping-A European LeukemiaNet WP10 study.
Leukemia. 2016;30:1769-1772. https://doi.org/10.1038/leu.2016.44 PMid:26922887
- Porwit
A, van de Loosdrecht AA, Bettelheim P, et al. Revisiting guidelines for
integration of flow cytometry results in the WHO classification of
myelodysplastic syndromes- proposal from the International/European
LeukemiaNet Working Group for Flow Cytometry in MDS. Leukemia.
2014;28:1793-1798. https://doi.org/10.1038/leu.2014.191 PMid:24919805
- van
de Loosdrecht AA, Ireland R, Kern W, et al. Rationale for the clinical
application of flow cytometry in patients with myelodysplastic
syndromes: position paper of an International Consortium and the
European LeukemiaNet Working Group. Leuk Lymphoma. 2013;54:472-475. https://doi.org/10.3109/10428194.2012.718341 PMid:22916713
- Ogata K, Satoh C, Hyodo H, et al. Association between
phenotypic features of blasts and the blast percentage in bone marrow
of patients with myelodysplastic syndromes. Leuk Res 2004;28:1171-1175
https://doi.org/10.1016/j.leukres.2004.0014 PMid:15380341
- Ogata
K, Kishikawa Y, Satoh C, et al. Diagnostic application of flow
cytometric characteristics of CD34+ cells in low-grade myelodysplastic
syndromes. Blood. 2006;108:1037-1044 https://doi.org/10.1182/blood-2005-12-4916 PMid:16574954
- Sternberg
A, Killick S, Littlewood T, et al. Evidence for reduced B-cell
progenitors in early (low-risk) myelodysplastic syndrome. Blood
2005;106:2982-2991. https://doi.org/10.1182/blood-2005-04-1543 PMid:16076868
- Maftoun-Banankhah
S, Maleki A, Karandikar NJ, et al. Multiparameter flow cytometric
analysis reveals low percentage of bone marrow hematogones in
myelodysplastic syndromes. Am J Clin Pathol 2008;129:300-308. https://doi.org/10.1309/4W2G3NDXUPG5J33N PMid:18208811
- Della
Porta MG, Malcovati L, Rigolin GM, et al. Immunophenotypic, cytogenetic
and functional characterization of circulating endothelial cells in
myelodysplastic syndromes. Leukemia. 2008;22:530-537. https://doi.org/10.1038/sj.leu.2405069 PMid:18094717
- Ogata
K, Della Porta MG, Malcovati L, et al. Diagnostic utility of flow
cytometry in low- grade myelodysplastic syndromes: A prospective
validation study. Haematologica 2009;94:1066-1074. https://doi.org/10.3324/haematol.2009.008532 PMid:19546439 PMCid:PMC2719029
- Della
Porta MG, Picone C, Pascutto C, et al. Multicenter validation of a
reproducible flow cytometric score for the diagnosis of low-grade
myelodysplastic syndromes: results of a European LeukemiaNET study.
Haematologica. 2012;97:1209-1217. https://doi.org/10.3324/haematol.2011.048421 PMid:22315489 PMCid:PMC3409819
- Bardet
V, Wagner-Ballon O, Guy J, et al. Multicentric study underlining the
interest of adding CD5, CD7 and CD56 expression assessment to the flow
cytometric Ogata score in myelodysplastic syndromes and
myelodysplastic/myeloproliferative neoplasms. Haematologica.
2015;100:472-478. https://doi.org/10.3324/haematol.2014.112755 PMid:25637056 PMCid:PMC4380720
- Loken
MR, Shah VO, Dattilio KL, Civin CI. Flow cytometric analysis of human
bone marrow: I. Normal erythroid development. Blood 1987;69:255-263
PMid:2947644
- Della
Porta MG, Malcovati L, Invernizzi R, et al. Flow cytometry evaluation
of erythroid dysplasia in patients with myelodysplastic syndrome.
Leukemia 2006;20:549-555 https://doi.org/10.1038/sj.leu.2404142 PMid:16498394
- Mathis
S, Chapuis N, Debord C, et al. Flow cytometric detection of
dyserythropoiesis: a sensitive and powerful diagnostic tool for
myelodysplastic syndromes. Leukemia. 2013;27:1981-1987. https://doi.org/10.1038/leu.2013.178 PMid:23765225
- Cremers
EM, Westers TM, Alhan C, et al. Implementation of erythroid lineage
analysis by flow cytometry in diagnostic models for myelodysplastic
syndromes. Haematologica. 2016, in press
- Westers
TM, Cremers EM, Oelschlaegel U, et al. Immunophenotypic analysis of
erythroid dysplasia in myelodysplastic syndromes. A report from the
IMDSFlow working group. Haematologica. 2016, in press
[TOP]