Kalman Filanovsky1*, Michal Haran1*, Vita Mirkin1, Andrei Braester2, Olga Shevetz1, Anfisa Stanevsky1, Erica Sigler1, Ekaterina Votinov1, Yehudit Zaltsman-Amir3, Alain Berrebi1, Atan Gross3 and Lev Shvidel1
1 Hematology Institute, Kaplan medical center, Rehovot, Israel, affiliated with the Hebrew University, Jerusalem, Israel.
2 Hematology institute, Galilee medical center, Nahariya, Israel.
3 Dept. of Biological Regulation, Weizmann Institute of Science, Rehovot, Israel.
* Both authors equally contributed to this manuscript.
Published: November 1, 2020
Received: June 20, 2020
Accepted: October 3, 2020
Mediterr J Hematol Infect Dis 2020, 12(1): e2020069 DOI
10.4084/MJHID.2020.072
This is an Open Access article distributed
under the terms of the Creative Commons Attribution License
(https://creativecommons.org/licenses/by-nc/4.0),
which permits unrestricted use, distribution, and reproduction in any
medium, provided the original work is properly cited.
|
Abstract
Structural
mitochondrial abnormalities and genetic aberrations in mitochondrial
proteins have been known in Myelodysplastic syndrome (MDS), yet there
is currently little data regarding MDS's metabolic properties and
energy production cells. In the current study, we used state-of-the-art
methods to assess OXPHOS in peripheral blood cells obtained from MDS
patients and healthy controls. We then assessed the effect of food
supplements- Coenzyme Q10 and carnitine on mitochondrial function and
hematological response. We show here for the first time that there is a
significant impairment of mitochondrial respiration in peripheral blood
cells in low-risk MDS, which can be improved with food supplements. We
also show that these supplements may improve the cytopenia and quality
of life.
|
Introduction
Normal
hematopoiesis is a well-coordinated process in which cell
proliferation, maturation, and apoptosis are tightly regulated in the
bone marrow.[1] In recent years, there is a growing
understanding of the importance of the tight control of the metabolic
program and the balance of glycolysis and mitochondrial oxidative
phosphorylation (OXPHOS) in maintaining quiescence and ensuring
adequate and timely maturation of hematopoietic stem cells.[2]
This growing understanding is mostly due to the development of better
tools to assess oxygen consumption rate (OCR) such as the Seahorse XF
analyzer (Agilent), which allows much more accurate OCR measurements
under basal conditions and also under conditions that force the cell to
utilize its spare respiratory capacity.
Myelodysplastic syndrome
(MDS) is a heterogeneous clonal disease of the bone marrow which is
manifested by ineffective hematopoiesis and genomic instability leading
to worsening cytopenias and a high percentage of transformation to
acute myeloid leukemia (AML).[3] The treatment options in MDS are quite limited.[4,5]
There
are several lines of evidence suggesting the importance of
mitochondrial dysfunction in the pathogenesis of MDS. First, structural
mitochondrial abnormalities have been known for many years;[6]
Second, the importance of genetic aberrations in mitochondrial proteins
in the prognosis of MDS and AML has been demonstrated by numerous
studies looking at the gene and protein expression.[7-8]
A recent study demonstrated early shedding of mitochondria from
reticulocytes in a sub-group of MDS patients, showing impaired
mitochondrial dynamics.[9] However, there is
currently little data regarding the metabolic properties and energy
production of peripheral blood MDS cells. We thought that in view of
treatment options that can improve mitochondrial energy production, it
is crucial to develop such an assay.
Such a readily available and inexpensive option is Coenzyme Q10 (CoQ10).
This vitamin-like compound is an essential component of the
mitochondrial electron transport chain and plays a central role in
mitochondrial oxidative phosphorylation and adenosine triphosphate
(ATP) production. Significant improvement in mitochondrial respiration
with a clinical improvement has been achieved by oral administration of
therapeutic doses of CoQ10 in various diseases.[10,11]
Further support for the role of impairment of mitochondrial function in
the pathogenesis of the disease as well as a possible novel therapeutic
approach was seen in a pilot study on low-risk MDS patients in which
treatment with CoQ10 resulted in about 25% hematologic response and even cytogenetic response in some of the patients.[12]
L-carnitine
is required to transport fatty acids from the cytosol to the
mitochondrial during the breakdown of lipids. It has also been used to
improve mitochondrial function and has been shown to have a synergistic
effect when combined with coenzyme Q10 in various medical conditions.[13–16]
Patients and methods
Clinical trial design.
This was an open-label, investigator-initiated study. Inclusion
criteria: low to intermediate 1 MDS according to IPSS, not
requiring or not eligible for treatment other than supportive care, and
having at the onset at least one of the following: Hgb<10.5g/dL,
PLT<100x109/L or ANC<0.8X109/L.
Concomitant erythropoietin-stimulating agents (ESAs) were permitted as
long as patients were treated for at least twelve weeks without further
hematological improvement. A minor effect (such as the need for blood
transfusion every ten days instead of every week was allowed). The
reason for this, was because we thought it was unethical to withhold
treatment that the patients felt was beneficial to them.
For
optimal enhancement of mitochondrial function, patients were given a
combination of Ultrasome™ (a better-absorbed formulation of CoQ10)-180
mg/day, L-carnitine-2000 mg/day, and a standard vitamin-mineral complex
to ensure that there will be no lack of essential vitamins or
minerals (a kind donation of Herbamed LTD, Israel), to be taken
daily for six months. Patients were allowed to continue treatment for
an additional six months if they desired to do so, and it was deemed
beneficial by the protocol committee. Quality of life (QOL) was
assessed using the FACT questionnaire[17] at the
beginning and third-month endpoint. We thought it was important to
assess patient-reported outcomes alongside the objective parameters.[18]
Samples for assessing in vitro mitochondrial energy production in
peripheral blood cells were taken in some of the patients before and
after six months of treatment.
Endpoints were: hematological response according to IWG criteria[3]
and meaningful improvement in the quality of life and functional
ability after three months of treatment with food supplements.
The study was approved by local IRB and registered at the National Institute of Health (NIH), registry no. NCT02042482.
Assessment of mitochondrial function. Mitochondrial function was assessed in peripheral blood cells using the Seahorse XF Analyzer, as previously described.[19]
The XF Analyzer creates micro-chambers of only a few microliters in
specialized cell culture micro¬plates. This enables OCR (oxygen
consumption rate) to be monitored in real-time. Briefly, cells were
plated on a Cell-Tak™ coated 24 well XF V7 cell culture microplate at
0.5×106 cells per well in 50 μL of XF
assay medium. Plates were centrifuged at 1200 rpm for 5 minutes to
allow the cells to adhere. 450 ul XF assay medium containing 5mM
glucose, 2mM pyruvate, and 2 mM Glutamax (Invitrogen) at pH 7.4 were
added to each well. Cells were incubated for one hour without CO2 for equilibration before XF bioenergetic assay. Basal Oxygen Consumption Rate (OCR: picomoles of O2
per minute) was measured, followed by consecutive injections of the
following: 1. Oligomycin (ATP synthase inhibitor); 2. the electron
transport chain accelerator FCCP (5 µM
gives the maximal OCR capacity of the cells); 3. The electron
transport-chain-inhibitor rotenone and Antimycin A, which inhibit
mitochondrial respiration.
We decided to perform all experiments
on fresh peripheral blood cells, which were used to avoid the need for
repeat bone marrow aspirations. This was based on the fact that since
previous studies have shown that peripheral blood cells harbor the same
mutations as less mature cells obtained from the bone marrow,[20]
thus making it likely that peripheral blood cells will harbor the same
genetic aberrations which affect mitochondrial respiration. Blood cells
were not separated, and we know that mostly neutrophils and platelets
could contribute to the results.
In each experiment, samples
from MDS patients were compared to samples obtained from matched
volunteers without hematological disorders to control for inter-assay
variability. The same controls were used for all samples obtained from
the same patient.
Statistical analysis. Statistical analysis was performed using the paired student t-test. The significance level was set at p<0.05.
Results
Clinical effect of food supplements. From May 2013 to April 2017, 33 MDS patients were enrolled in the clinical trial (Table 1).
There were 20 males and 13 females, with a median age of 75 years
(range: 56-93). The IPSS scoring showed a low risk in 19 (58%) and
intermediate-1 risk in 14 (42%) patients; 13 patients (39%) had MDS
with ring sideroblasts (MDS-RS). The median Hb level was 9.5 g/dL
(range: 6.4-12.2). Seventeen patients (52%) were
transfusion-dependent, requiring an average of 2.0 (range, 0.5-10)
packed cell infusions per month. Twenty-eight patients completed the
treatment protocol. Six patients (21.4%) achieved hematological
improvement according to IWG criteria (four of these were previously
transfusion-dependent, and one showed a bi-lineage response (see Figure 1
for a representative case). In 19 patients (67.8%) there was a stable
disease at the end of the study. Interestingly, the hematological
response in MDS-RS was similar to that seen in other MDS subtypes. The
median time to initial response was nine weeks (range, 5-24), and the
median duration of hematological improvement in responders was 54.5
weeks (range: 10-212).
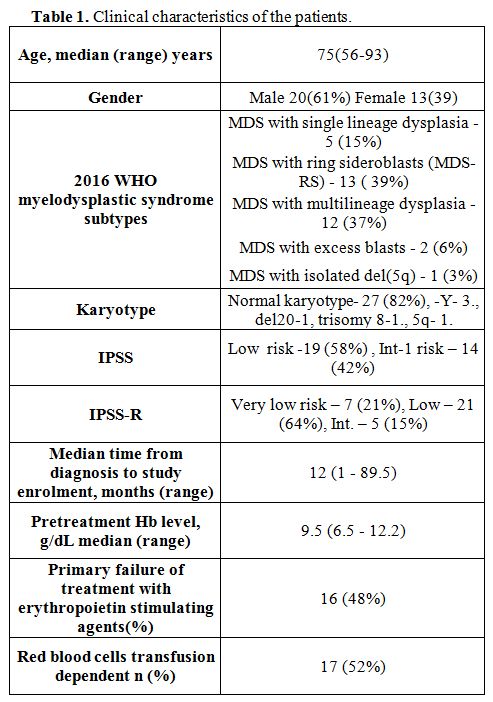 |
Table 1. Clinical characteristics of the patients. |
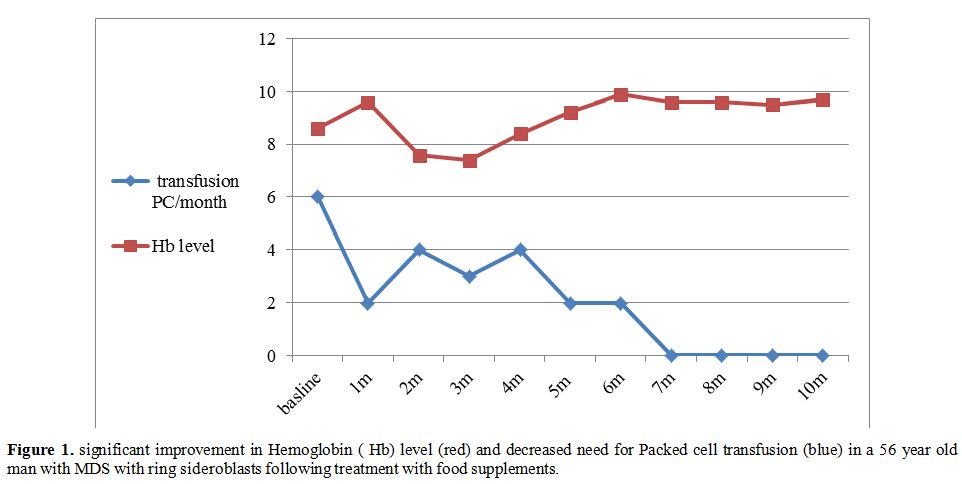 |
Figure 1 Significant improvement in
Hemoglobin (Hb) level (red) and decreased need for Packed cell
transfusion (blue) in a 56 year old man with MDS with ring sideroblasts
following treatment with food supplements. |
The
FACT questionnaire was administered to 18 patients before and three
months endpoint. There was a significant improvement in the mean FACT
score (126.3±26.9 points before treatment vs. 117.3±26.3 after
treatment; p=0.011). Notably, the scores' improvement was mostly in the
physical well-being domains (mean, 22.2 points vs. 18.5; p=0.03) and
not in the social and emotional domains. Interestingly These results
demonstrated a moderate, though significant improvement in overall
physical ability and quality of life, even in those patients that did
not have a discernible hematological response.
Peripheral
blood cells obtained from MDS patients have significant impairment of
mitochondrial respiration, which can be reversed with food supplements.
Mitochondrial oxidative phosphorylation was assessed in peripheral
blood cells obtained from 20 MDS patients. Each experiment was done on
a patient matched to a healthy control and repeated three times. In
most patients (18/20), there was a significant decrease in basal oxygen
consumption rate and the spare respiratory capacity (see Figure 2a for a representative patient) compared to healthy controls. This result was statistically significant (see Figure 2b). Interestingly, there was an increased oxygen consumption rate in two patients with excess of blasts in bone marrow (see Figure 3 for a representative patient).
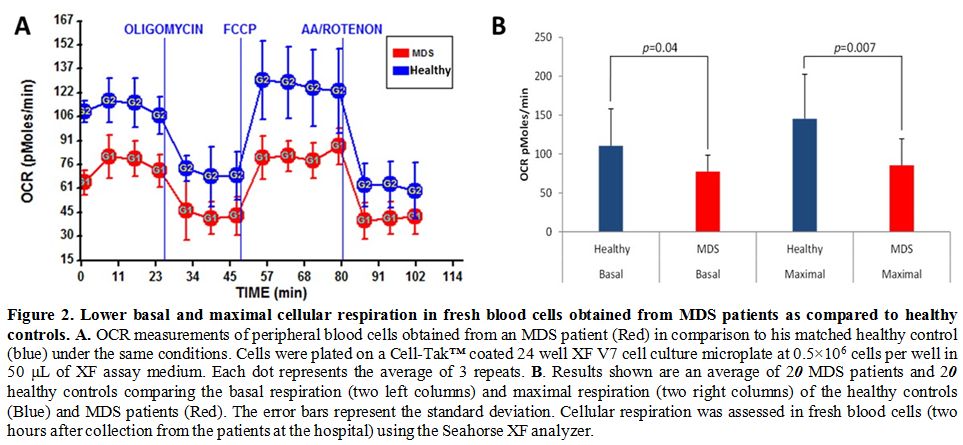 |
Figure 2. Lower basal and maximal cellular respiration in fresh blood cells obtained from MDS patients as compared to healthy controls. A.
OCR measurements of peripheral blood cells obtained from an MDS patient
(Red) in comparison to his matched healthy control (blue) under the
same conditions. Cells were plated on a Cell-Tak™ coated 24 well XF V7
cell culture microplate at 0.5×106 cells per well in 50 μL of XF assay medium. Each dot represents the average of 3 repeats. B. Results
shown are an average of 20 MDS patients and 20 healthy controls
comparing the basal respiration (two left columns) and maximal
respiration (two right columns) of the healthy controls (Blue) and MDS
patients (Red). The error bars represent the standard deviation.
Cellular respiration was assessed in fresh blood cells (two hours after
collection from the patients at the hospital) using the Seahorse XF
analyzer. |
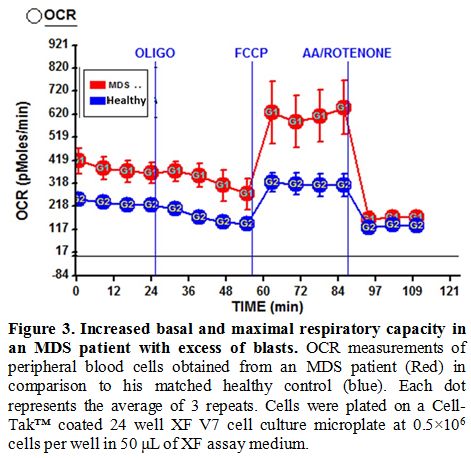 |
Figure 3 Increased basal and maximal respiratory capacity in an MDS patient with excess of blasts.
OCR measurements of peripheral blood cells obtained from an MDS patient
(Red) in comparison to his matched healthy control (blue). Each dot
represents the average of 3 repeats. Cells were plated on a Cell-Tak™
coated 24 well XF V7 cell culture microplate at 0.5×106 cells per well in 50 μL of XF assay medium. |
After
six months of treatment, OCR assessment was done in five patients
matched to their previous controls. The value pre-treatment was
compared to the value post-treatment. A discernible improvement was
seen in all the patients tested. The difference in OCR pre and
post-treatment was statistically significant (p<0.05). (Figure 4).
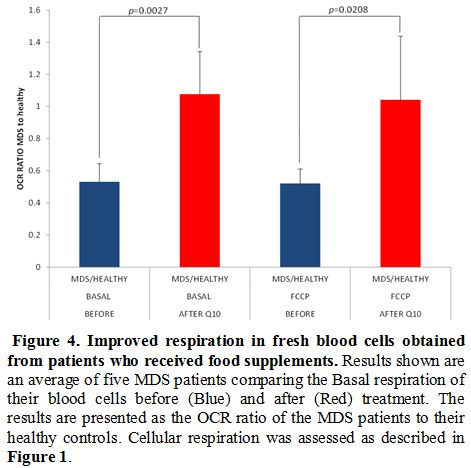 |
Figure 4. Improved respiration in fresh blood cells obtained from patients who received food supplements.
Results shown are an average of five MDS patients comparing the Basal
respiration of their blood cells before (Blue) and after (Red)
treatment. The results are presented as the OCR ratio of the MDS
patients to their healthy controls. Cellular respiration was assessed
as described in Figure 1.
|
Safety and tolerability of combination supplement treatment.
The treatment with food supplements was well tolerated; most adverse
events were transient gastrointestinal symptoms of grade 2 or less.
Surprisingly, two patients who had more advanced disease with an excess
of blasts in the bone marrow developed disease progression within the
first few weeks of treatment. Those patients also had increased OCR
before treatment. Interestingly, it was shown that AML cells had
increased OCR,[21] suggesting that increased OXPHOS
may be the first step in developing AML. As we could not rule out the
possibility that the food supplements augmented progression, we amended
the protocol to exclude patients with excess (over 5%) of blasts.
Another unexpected observation involved two patients who developed
gastric diffuse large cell lymphoma one month and 30 months after
cessation of treatment.
Discussion
The importance of mitochondrial energy metabolism is well recognized in normal hematopoiesis.[2]
Impairment of mitochondrial function in MDS has been strongly implied
by the combination of structural mitochondrial abnormalities and early
reticulocyte mitochondrial shedding present in some sub-groups,[9] as well as abnormalities in genes essential for mitochondrial function.[8]
However, such impairment of mitochondrial energy production was never
demonstrated directly in peripheral blood cells. A previous study
suggested a significantly impaired OCR in the bone marrow, but not in
peripheral blood cells that harbor the same mutations.[22]
The discrepancy between this study and ours could be the inability of
the Clark electrode used in that study to differentiate between
different types of OCR.
In the current study,
utilizing novel tools, we directly show for the first time that
mitochondrial energy production is indeed impaired in peripheral blood
cells obtained from patients with low-risk MDS. We showed that both the
basal OCR and the spare respiratory capacity were significantly
decreased compared to age-matched healthy controls. Those finding could
have both diagnostic and therapeutic implications. It may also provide
a non-invasive tool for monitoring response to treatment. Furthermore,
we showed that this impairment in mitochondrial respiration can be
partially reversed with food supplementations known to augment
mitochondrial function. In some of the patients, this was also
accompanied by a hematological response.
Galili et al. demonstrated a clinical benefit of high dose CoQ10 in some MDS patients.[12] Likewise, combined treatment with CoQ10
and carnitine ameliorated anemia and RBC-transfusion-dependence in 6
out of 28 (21.4%) in our patient group. This improvement is noteworthy,
as generally, low-risk MDS patients have minimal treatment options once
they do not respond to ESAs. Interestingly, although the hematological
response was achieved only in some of the patients treated with
supplements, patient-reported outcomes of overall strength and physical
ability were increased in most of them, along with improvement in
mitochondrial respiration of peripheral blood cells.
The
treatment was generally well tolerated. An unexpected observation,
which is not in line with the reported side effects of the used food
supplements, was a relatively high prevalence of second hematological
malignancies in our cohort. It is hard to discern in such a small
series of patients whether this is related to the treatment or
coincidental with it. Progression to AML in MDS patients is frequent;
also, lymphoid malignancies may be more prevalent than in the general
population, and gastric lymphoma cases in patients with MDS have been
described.[23] Moreover, it has been shown that peripheral blood lymphocytes obtained from MDS patients harbor pre-malignant mutations.[24]
Overall, this treatment seems to be safe and effective in most low-risk
MDS patients. Caution should be taken in patients with excess of
blasts. This finding is important as many times patients take food
supplements without the awareness of their clinicians.
Our
study's limitation was that it was not randomized and that food
supplements were given for a relatively short period. Further studies
with a much longer follow-up are required to assess the safety and
efficacy, as well as timing of treatment with the food supplements in
different sub-groups of MDS patients.
Acknowledgments
We are grateful to Haim Aviv PhD for his help in the design of the research and donation of the food supplements.
Funding
This study was funded by a grant from the Rising Tide Foundation and Israel Society of Hematology and Blood transfusion.
References
- Maryanovich M, Oberkovitz G, Niv H, et al. The
ATM-BID pathway regulates quiescence and survival of haematopoietic
stem cells. Nat Cell Biol 2012;14(5):535-41. https://doi.org/10.1038/ncb2468 PMid:22446738
- Haran
M, Gross A. Balancing glycolysis and mitochondrial OXPHOS: lessons from
the hematopoietic system and exercising muscles. Mitochondrion 2014;19
Pt A3-7. https://doi.org/10.1016/j.mito.2014.09.007 PMid:25264322
- Kulasekararaj
AG, Mohamedali AM, Mufti GJ. Recent advances in understanding the
molecular pathogenesis of myelodysplastic syndromes. Br J Haematol
[Epub ahead of print].
- Greenberg PL, Sun
Z, Miller KB, et al. Treatment of myelodysplastic syndrome patients
with erythropoietin with or without granulocyte colony-stimulating
factor: results of a prospective randomized phase 3 trial by the
Eastern Cooperative Oncology Group (E1996). Blood
2009;114(12):2393-2400. https://doi.org/10.1182/blood-2009-03-211797 PMid:19564636 PMCid:PMC2746469
- Fenaux
P, Santini V, Spiriti MAA, et al. A phase 3 randomized,
placebo-controlled study assessing the efficacy and safety of epoetin-α
in anemic patients with low-risk MDS. Leukemia 2018;32(12):2648-2658. https://doi.org/10.1038/s41375-018-0118-9 PMid:29895954 PMCid:PMC6286328
- van
de Loosdrecht AA, Brada SJ, Blom NR, et al. Mitochondrial disruption
and limited apoptosis of erythroblasts are associated with high risk
myelodysplasia. An ultrastructural analysis. Leuk Res
2001;25(5):385-93. https://doi.org/10.1016/S0145-2126(00)00151-X
- Gattermann
N. From sideroblastic anemia to the role of mitochondrial DNA mutations
in myelodysplastic syndromes. Leuk Res 2000;24(1999):141-151. https://doi.org/10.1016/S0145-2126(99)00160-5
- Schildgen
V, Wulfert M, Gattermann N. Impaired mitochondrial gene transcription
in myelodysplastic syndromes and acute myeloid leukemia with
myelodysplasia-related changes. Exp Hematol 2011;39(6):666-675.e1. https://doi.org/10.1016/j.exphem.2011.03.007 PMid:21447369
- Zhang
Q, Steensma DP, Yang J, Dong T, Wu MX. Uncoupling of CD71 shedding with
mitochondrial clearance in reticulocytes in a subset of myelodysplastic
syndromes. Leukemia 2019;33(1):217-229. https://doi.org/10.1038/s41375-018-0204-z PMid:30050123 PMCid:PMC7432991
- Nicolson
GL, Conklin ÆKA. Reversing mitochondrial dysfunction , fatigue and the
adverse effects of chemotherapy of metastatic disease by molecular
replacement therapy. Clin Exp Metastasis 2008;161-169. https://doi.org/10.1007/s10585-007-9129-z PMid:18058028
- Quinzii CM, Hirano M. Coenzyme Q and mitochondrial disease. Dev Disabil Res Rev 2010;16(2):183-188. https://doi.org/10.1002/ddrr.108 PMid:20818733 PMCid:PMC3097389
- Galili
N, Sechman E V, Cerny J, et al. Clinical response of myelodysplastic
syndromes patients to treatment with coenzyme Q10. Leuk Res
2007;31(1):19-26. https://doi.org/10.1016/j.leukres.2006.04.009 PMid:17064768
- Lee
JH, Kim MJ, Park SH, Chae JH, Shin K. Case study of an inborn error
manifested in the elderly: A woman with adult-onset mitochondrial
disease mimicking systemic vasculitis. Int J Rheum Dis
2019;22(6):1152-1156. https://doi.org/10.1111/1756-185X.13575 PMid:30968563
- Lowry
E, Marley J, McVeigh JG, McSorley E, Allsopp P, Kerr D. Dietary
Interventions in the Management of Fibromyalgia: A Systematic Review
and Best-Evidence Synthesis. Nutrients;12(9):. https://doi.org/10.3390/nu12092664 PMid:32878326
- Hajihashemi
P, Askari G, Khorvash F, Reza Maracy M, Nourian M. The effects of
concurrent Coenzyme Q10, L-carnitine supplementation in migraine
prophylaxis: A randomized, placebo-controlled, double-blind trial.
Cephalalgia 2019;39(5):648-654. https://doi.org/10.1177/0333102418821661 PMid:30612463
- Hirschey
MD, Shimazu T, Goetzman E, et al. SIRT3 regulates mitochondrial
fatty-acid oxidation by reversible enzyme deacetylation. Nature
2010;464(7285):121-5. https://doi.org/10.1038/nature08778 PMid:20203611 PMCid:PMC2841477
- Brandenburg
NA, Yu R, Revicki DA. Reliability and Validity of the FACT-AN In
Patients with Low or Int-1-Risk Myelodysplastic Syndromes with Deletion
5q. Blood;116(21). https://doi.org/10.1182/blood.V116.21.3827.3827
- Mercieca-Bebber
R, King MT, Calvert MJ, Stockler MR, Friedlander M. The importance of
patient-reported outcomes in clinical trials and strategies for future
optimization [Epub ahead of print].
- Mufti
GJ, Bennett JM, Goasguen J, et al. Diagnosis and classification of
myelodysplastic syndrome: International Working Group on Morphology of
myelodysplastic syndrome (IWGM-MDS) consensus proposals for the
definition and enumeration of myeloblasts and ring sideroblasts.
Haematologica 2008;93(11):1712-7. https://doi.org/10.3324/haematol.13405 PMid:18838480
- A.M.
M, J. G, M. A, et al. Comparison of peripheral blood and bone marrow
molecular profiling in primary Myelodysplastic Syndromes (MDS). Blood
[Epub ahead of print].
- Sriskanthadevan
S, Jeyaraju D V, Chung TE, et al. MYELOID NEOPLASIA AML cells have low
spare reserve capacity in their respiratory chain that renders them
susceptible to oxidative metabolic stress. 2015;125(13):2120-2131. https://doi.org/10.1182/blood-2014-08-594408 PMid:25631767 PMCid:PMC4375109
- Bowen
D, Peddie C. Mitochondrial oxygen consumption and ineffective
haematopoiesis in patients with myelodysplastic syndromes. Br J
Haematol 2002;118(1):345-346. https://doi.org/10.1046/j.1365-2141.2002.03576_2.x PMid:12100173
- Dozzo
M, Zaja F, Volpetti S, et al. Two cases of concomitant diffuse large
B-cell lymphoma and myelodysplastic syndrome. Am J Hematol
2014;89(10):1011-1013. https://doi.org/10.1002/ajh.23783 PMid:24912474
- Shlush LI. Change comes like a little wind: tales in MDS evolution. Blood 2016;128(9):1162-3. https://doi.org/10.1182/blood-2016-07-722660 PMid:27587865
[TOP]