XiaoTian Zhang1, Yanhui Yu1, Chao Zhang2, Hongrui Wang3, Lijuan Zhao3, Hua Wang3, Yingying Su4* and Ming Yang3*.
1 Department of Clinical Laboratory, The second Hospital of Jilin University, Changchun, Jilin, China.
2 Department of Nuclear Medicine,The second Hospital of Jilin University, Changchun, Jilin, China.
3 Department of Molecular Biology, College of Basic Medical Sciences, Jilin University, Changchun 130021, Jilin Province, China.
4 Department of Anatomy, College of Basic Medicine Sciences of Jilin University, Jilin, China.
Correspondence to:
Ming Yang, phD, Associated Professor, Department of Molecular Biology,
College of Basic Medical Sciences, Jilin University, No.126, Xinmin
Street, Chaoyang District, Changchun 130021, Jilin Province, China.
E-mail:
myang48@jlu.edu.cnYingying
Su, phD, Lecturer, Department of Anatomy, College of Basic Medicine
Sciences of Jilin University, Jilin, China. E-mail:
suyingying@jlu.edu.cn
Published: January 1, 2022
Received: July 23, 2021
Accepted: November 23, 2021
Mediterr J Hematol Infect Dis 2022, 14(1): e2022003 DOI
10.4084/MJHID.2022.003
This is an Open Access article distributed
under the terms of the Creative Commons Attribution License
(https://creativecommons.org/licenses/by-nc/4.0),
which permits unrestricted use, distribution, and reproduction in any
medium, provided the original work is properly cited.
|
Abstract
Many
studies have shown that patients with Coronavirus disease 2019
(COVID-19) have different degrees of liver injury. However, the
mechanisms of severe acute respiratory syndrome coronavirus 2
(SARS-CoV-2) invasion into the liver are still not fully understood.
This review mainly summarizes the recently published works on the
abnormal liver biochemical indicators and the mechanism of viral
invasion with liver injury in COVID-19 patients. Generally, SARS-CoV-2
infection of the liver was caused by blood circulation or retrograde
infection of the digestive tract, which led to the liver injury through
direct cytopathic effect induced by virus or immunopathological effect
caused by excessive inflammation. Besides these, hypoxia, endothelial
injury and drug-induced jury were also the main reasons of liver injury
in COVID-19 patients. In the liver function indicators, elevated
alanine aminotransferase, aspartate aminotransferase, alkaline
phosphatase, gamma-glutamyl transpeptidase, and lactate dehydrogenase
levels with reduced albumin levels were observed in COVID-19 patients.
|
Introduction
The
coronavirus disease 2019 (COVID-19) outbreak, which is caused by severe
acute respiratory syndrome coronavirus 2 (SARS-CoV-2), has become a
global public health problem. As of July 15, 2021, SARS-CoV-2 infection
had led to a total of 189,219,660 confirmed cases and 4,071,640 deaths
documented in 219 countries. SARS-CoV-2 mainly comprises four
structural proteins: the spike (S), the membrane, the envelope, and the
nucleocapsid protein.[1] The S protein recognizes the receptor on the host cell surface and has pivotal roles in viral infection and pathogenesis.
It
is well established that most individuals with COVID-19 present with
fever and typical respiratory symptoms. However, abnormal liver
function is often found in patients with COVID-19. With the increase in
cases and further research, clinical studies suggest that liver
biochemical indicators, such as serum alanine aminotransferase (ALT),
aspartate aminotransferase (AST), gamma-glutamyltransferase (GGT) and
alkaline phosphatase (ALP) were significantly increased in COVID-19
patients, which suggests hepatocellular and cholangiocellular damage.[2] Patients with liver damage are at higher risk of developing severe disease.[3] Therefore, clinicians should be alert to the possibility of liver injury in COVID-19 patients.
The
major pathophysiological features of liver injury include enlargement
of liver volume, liver cell focal necrosis with neutrophil
infiltration, hepatocyte steatosis, lobular and portal inflammation,
hepatic sinus congestion, microthrombosis, and high filling of the
gallbladder.[4] These pathological changes in the
liver suggested that the degree of liver injury in most COVID-19
patients was mild and that abnormal liver function might be caused by a
viral infection, drug-induced or immune-mediated liver injury. However,
it is still unknown how does the virus invade the liver and whether
increased serum liver biochemistry is caused by hepatic injury or
systemic progression of the disease. Therefore, it is crucial to
understand the routes and mechanism of viral invasion to the liver and
the effect of liver biochemistry abnormalities on the progression and
prognosis of COVID-19. This review will highlight the process of virus
invasion in the liver and the pathogenesis of liver injury, explain the
changes in liver biochemistry after COVID-19 infection, and provide a
theoretical basis for clinical evaluation of liver injury in COVID-19
patients.
Routes of Viral Invasion to Liver
Receptors of SARS-CoV-2 in Liver.
ACE2 (angiotensin-converting enzyme 2) receptor, an important part of
the renin-angiotensin-aldosterone system, is the gateway for SARS-CoV-2
to enter host cells. SARS-CoV-2 binds to the ACE2 receptor through its
surface S protein,[5] which is similar to SARS-CoV,
but the binding affinity of SARS-CoV-2 to ACE2 receptor is 10-20 times
higher than that of SARS-CoV.[6] Many studies analyzed
the distribution of ACE2 receptors in different tissues and cell types
of the human body using immunohistochemistry, RNA sequencing and other
methods. The results showed that the tissues with the highest
expression of ACE2 receptor were: small intestine, colon, duodenum,
kidney, testis, gallbladder. On the other hand, the distribution of
receptors in the liver is low, mainly expressed in bile duct cells.[7-9]
Interestingly, the expression of ACE2 in the lung is lower than that in liver,[7]
mainly in alveolar type 2 epithelial cells, but the lung is recognized
as the primary site of viral invasion. Thus, the distribution of ACE2
receptors is not completely consistent with the load of virus in
organs. Studies also suggest that ACE2 is abundant in endothelial cells
and smooth muscle cells of almost all organs.[10]
Therefore, once the virus enters the blood circulation, it is easy to
spread in the body. Generally, the presence of virus particles in the
liver confirmed the possibility of direct virus infection in the liver,
but it is not clear whether the virus directly invades the liver
through the ACE2 receptor.
Blood Circulation Transmission of SARS-Cov-2.
With a large number of viral SARS-CoV-2 replication and an accumulation
of a variety of pro-inflammatory cytokines and chemokines, the alveolar
epithelial cells were damaged, and the integrity of the air-blood
barrier was seriously interrupted.[11] In addition to
alveolar epithelial cells, SARS-CoV-2 can also invade pulmonary
capillary endothelial cells, resulting in a large amount of plasma
components exudate in the alveolar cavity; as shown in Figure 1.
Since the alveoli are located at the end of the respiratory tract and
contain an abundant capillary network, SARS-CoV-2 can diffuse from the
damaged alveoli into the capillaries and spread through the blood
circulation. In clinical cases, sepsis and septic shock were found in
many COVID-19 patients with a severe and critical illness. However,
bacterial and fungal cultures in blood and lower respiratory tract
specimens of 76% of septicemia patients were negative in 76% of
septicemia patients,[12] suggesting that the clinical
manifestations of septicemia were caused by viral infection. The liver
has a double and abundant blood flow, so it is easily affected by
COVID-19 in circulating blood. It has been found that SARS-CoV-2 virus
particles exist in the portal vein lumen and endothelial cells of liver
samples from patients with COVID-19,[13] which confirmed that the virus might infect the liver with blood circulation.
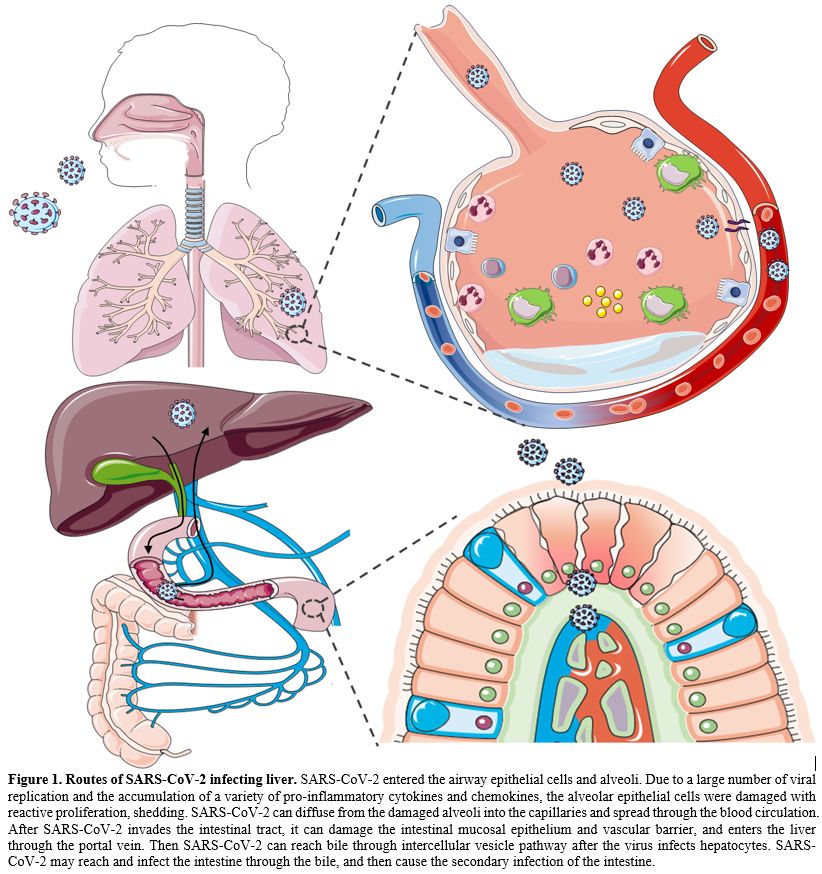 |
Figure
1. Routes of SARS-CoV-2 infecting liver. SARS-CoV-2 entered the airway epithelial cells and alveoli.
Due to a large number of viral replication and the accumulation of a
variety of pro-inflammatory cytokines and chemokines, the alveolar
epithelial cells were damaged with reactive proliferation, shedding.
SARS-CoV-2 can diffuse from the damaged alveoli into the capillaries
and spread through the blood circulation. After SARS-CoV-2 invades the
intestinal tract, it can damage the intestinal mucosal epithelium and
vascular barrier, and enters the liver through the portal vein. Then
SARS-CoV-2 can reach bile through intercellular vesicle pathway after
the virus infects hepatocytes. SARS-CoV-2 may reach and infect the
intestine through the bile, and then cause the secondary infection of
the intestine.
|
Retrograde Infection of the Digestive Tract in COVID-19 Patients.
In addition to the blood circulation, the spread of the virus in the
digestive tract has gradually attracted people's attention. Since the
ACE2 receptor is most widely distributed in the small intestine, colon
and duodenum, it provides convenience for SARS-CoV-2 infection. About
50% of the patients had fecal excretion of virus and could isolate
infectious SARS-CoV-2 particles from it. Moreover, viral nucleic acid
in feces remained positive for more than two weeks after the
respiratory system nucleic acid test turned negative.[14] At present, many studies have confirmed that COVID-19 can directly lead to gastrointestinal infection[15-17]
through biopsy of the duodenum and rectum, and that suggests that the
gastrointestinal tract is also the main site of SARS-CoV-2 infection.
Some patients with intestinal injury were more likely to have a severe liver injury than those with normal intestinal tract.[18]
Similarly, people with underlying liver diseases are at a higher risk
of developing intestinal manifestations after SARS-CoV-2 infection,
suggesting a relationship between gastrointestinal and liver injury.
Actually, there is a very important two-way communication pathway
between the intestine and the liver, called the "gut-liver axis".[19]
The liver secretes synthetic bile acids and other active substances
into the intestine, and the bacterial metabolites and nutrients in the
intestine can be absorbed into the blood through the portal vein and
then flow back to the liver.[20] Therefore, it is
speculated that there is a possibility of retrograde infection of the
liver after SARS-CoV-2 infection of the intestinal tract. After
SARS-CoV-2 invades the intestinal tract can damage the intestinal
mucosal epithelium and vascular barrier and carry out retrograde
infection through the portal vein. In addition, SARS-CoV-2 virus
particles can reach bile through the intercellular vesicle pathway
after the virus infects hepatocytes. Therefore, bile duct cells may
also contact and infect SARS-CoV-2. Because the biliary tract provides
a direct connection between the liver and the intestine, SARS-CoV-2 may
reach and infect the intestine through the bile and then cause the
secondary infection of the intestine, as shown in Figure 1. Molecular Mechanism of Liver Injury in COVID-19 Infection
Many
COVID-19 patients have been found to have different degrees of liver
injury, but the mechanism of liver injury remains unclear. The liver
plays an important role in host defense against microorganisms, and
some viruses can directly cause cytopathic effects on hepatocytes and
bile duct cells. In addition, hypoxia, vascular endothelial injury,
drug-induced and immunoinflammatory play a key role in the
pathophysiological mechanisms of liver injury in COVID‐19.
Direct Action of The Virus.
Although ACE2 expression levels are very low in the liver, the
distribution of the ACE2 receptor is not consistent with that of organ
infection.[10,21,22] A number of
studies have been conducted on liver samples from postmortem cases of
COVID-19 deaths. It was found that SARS-CoV-2 RNA can be detected in
the liver of COVID-19 patients.[23] Wang et al.[4]
performed liver biopsies on two dead COVID-19 patients with elevated
transaminase. Through transmission electron microscopy,
immunohistochemistry and pathological studies, it was found that there
were a large number of SARS-CoV-2 virus particles in the cytoplasm of
liver cells of the two COVID-19 patients. Most virus particles have an
intact envelope with a coronal process, suggesting that SARS-CoV-2 can
enter liver cells and replicate in them.
A series of studies
showed that SARS-CoV-2 infection in the liver directly contributes to
hepatic impairment in patients with COVID-19. The mitochondria of liver
cells infected with SARS-CoV-2 were swollen, the endoplasmic reticulum
was expanded, and the glycogen granules were reduced. Histologically, a
large amount of hepatocyte apoptosis and some binuclear hepatocytes
were observed. Ultrastructural and histological evidence shows a
typical viral infection lesion, and SARS-CoV-2 can directly damage the
liver and cause abnormal liver transaminase.[4] In
addition, in situ hybridizations of liver samples revealed that
SARS-CoV-2 virions were present in the vascular lumen and portal vein
endothelial cells of liver samples from COVID-19 patients.[13]
Approximately 2-10% of COVID-19 patients develop symptoms of diarrhea.
Nucleic acid of SARS-CoV-2 was detected in their stool and blood
samples, suggesting that the virus may invade the liver through the
digestive tract or the circulation.[24] However, the mechanisms of how infection with SARS-CoV-2 can directly lead to liver injury remains unclear.
ACE2
is lowly expressed in the liver, but it is highly expressed in the bile
duct. Thus, liver injury caused by SARS-CoV-2 infection may also derive
from the biliary tract system. Furthermore, studies have shown that
ACE2 can be expressed in bile duct endothelial cells with a specificity
of up to 59.7%, which is 20 times higher than the expression in liver
cells, suggesting that SARS-CoV-2 may further affect liver function and
bile excretion by directly binding to bile duct epithelial cells.[25] Using a system of human stem cells grown in vitro, Zhao's team[26]
constructed "liver ductal organoids". A large number of ACE2 receptors
were found in this organ, and it was highly susceptible to the
SARS-CoV-2 virus. Moreover, SARS-CoV-2 infection can reduce the
expression of the Claudin1 gene, leading to the destruction of the
barrier function of bile duct cells, resulting in bile duct cell damage
and the corresponding accumulation of bile acid and thus the symptoms
of liver injury.
Hypoxia and Vascular Endothelial Injury.
The liver is an important organ responsible for digestion and
metabolism. It has a complex dual blood supply system and is a highly
aerobic tissue and organ. An oxygen partial pressure gradient
phenomenon exists, and it is extremely sensitive to hypoxia. Patients
with COVID-19 have different degrees of hypoxemia, and hypoxia is a
typical feature of the disease cases. In addition, severe patients can
also be complicated with systemic inflammatory response syndrome,
respiratory distress syndrome, and multiple organ failure, worsening
liver tissue ischemia and hypoxia. Hepatic ischemia and hypoxia can
activate Kupffer cells, neutrophils, and platelets, causing a series of
destructive cellular responses and leading to inflammation and cell
damage.[27] Meanwhile, the microcirculation
disturbance caused by hepatic sinusoidal endothelial cell injury can
further aggravate hepatic ischemia and hypoxia. Hypoxia is an important
regulator of ACE2 and can upregulate its expression in hepatocytes.[28] The high expression of ACE2 in hepatocytes and bile duct endothelial cells may promote the entry of novel coronavirus.[29,30,31] These may explain why extrapulmonary transmission of SARS-COV-2 is mainly seen in ARDS and other hypoxic patients.
The
current view is that COVID-19 is a vascular disease with clotting
disorders and thrombosis. SARS-COV-2 can infect endothelial cells and
cause diffuse endothelitis. Subsequent microvascular dysfunction leads
to hypercoagulation, tissue edema, and organ ischemia.[32,33]
Diffuse intravascular coagulation caused by vascular endothelial cell
injury can also affect the blood supply to the liver, resulting in
hepatic microcirculation disorder and liver function impairment. In
addition, recent studies have found that the glycolytic pathway is
significantly enhanced to provide energy for virus survival and
replication after the virus infects host cells, thus mediating the
production of a large number of reactive oxygen species.[34]
Excessive reactive oxygen species can cause oxidative stress and lipid
peroxidation in liver cells, thus damaging the function of liver cells.[35]
Drug-Induced Liver Injury.
The liver is an important organ for drug transformation and metabolism.
These metabolites can lead to apoptosis or necrosis of liver cells
through cellular stress, destruction of mitochondrial membrane
permeability and specific immune responses.[36] At
present, common drugs such as antiviral, antibiotics and
immunoregulatory factors, glucocorticoid, sedative and other
proprietary Chinese medicines are used to treat COVID-19 patients,
leading to possible liver injuries.
A study found that ritonavir
led to a mild to moderate increase in transaminase expression in 44% of
COVID-19 patients. The main manifestation is cytolysis, but
cholestasis, steatosis, and fibrosis may also occur.[37,38] The drug is mainly metabolized by the liver cytochrome P4503A4 (CYP3A4) enzyme and can lead to elevated serum transaminase.[39]
Hydroxychloroquine caused mild to moderate transaminase elevation in
11% of COVID-19 patients: however, acute liver injury is rare and
sometimes accompanied by jaundice.[38,40]
Azithromycin causes mild to moderate transaminase elevation in 40% of
COVID-19 patients. A rare case of severe hepatotoxicity with hepatocyte
injury and jaundice or chronic cholestatic liver failure was reported.[41] Tocilizumab can cause transaminase elevation and acute liver injury in COVID-19 patients.[42] Glucocorticoids have also been shown to be associated with steatosis or glycogenic diseases.[43]
The mechanism of drug-induced hepatotoxicity mainly involves
mitochondrial dysfunction, oxidative stress, endoplasmic reticulum
stress, lipid dystrophy and insulin resistance.[44] Zhan et al.[45]
found that lopinavir/ritonavir was an independent risk factor for
severe liver injury in COVID-19 patients. Therefore, for patients with
decreased liver function, hepatotoxic drugs should be used with
caution. Cai et al. also found that lopinavir/ritonavir were the most
important risk factors for liver damage by analyzing the clinical case
characteristics and medication of 417 patients with COVID-19 after
admission. The use of lopinavir/ritonavir increased the risk of liver
damage by four times.[2] Yip's team analyzed liver
biochemistry data from 1040 COVID-19 patients at admission and during
hospitalization and found that the use of lopinavir/ritonavir,
ribavirin, interferon and glucocorticoids was independently associated
with increased ALT/AST in COVID-19 patients. Glucocorticoid use was
also associated with acute liver injury.[46] Thus, cautious use of antiviral agents in COVID-19 patients with decompensated liver disease should be considered.
Immunoinflammatory Injury.
With the increasing number of COVID-19 patients, it was found that the
clinical characteristics of some COVID-19 patients, not serious in the
early stage, could suddenly deteriorate rapidly and enter the state of
multiple organ failure, related to cytokine storms caused by excessive
immune responses in the body.[47] Cytokine storm
refers to the overactivation of the immune system caused by severe
stimulation, such as infection, which rapidly produces a large number
of cytokines, leading to a systemic inflammatory reaction with
mycrothrombosis,[48] which can induce hypoxia in the
body, leading to cell damage and necrosis. This process not only leads
to lung damage but also can involve the liver, heart, and kidney.
Current studies show that cytokine storm is an important node in the
transition from mild to severe and critical COVID-19 disease and an
important reason for the death of patients.[47] Serum
levels of pro-inflammatory cytokines, including interferon-α (IFN-α),
interferon-γ (IFN-γ), interleukin-1β (IL-1β), interleukin-2 (IL-2),
interleukin-6 (IL-6), interleukin-8 (IL-8), interleukin-10 (IL-10),
interleukin-18 (IL-18), interleukin-33 (IL-33), tumor necrosis factor-α
(TNF-α), granulocyte colony-stimulating factor (G-CSF),
granulocyte-macrophage colony-stimulating factor (GM-CSF),
interferon-inducible protein-10 (IP-10), and C-reactive protein (CRP),
were significantly increased in patients with severe COVID-19,[49] as shown in Figure 2. Huang et al.[47]
conducted a clinical summary of COVID-19 patients and found that 63% of
the patients had lymphocytopenia, and the plasma levels of IL-2, IL-7,
IL-10, G-CSF, IP-10, monocyte chemoattractant protein-1 (MCP-1),
macrophage inflammatory protein 1A (MIP1A) and TNF-α were higher in
critically ill patients. It is speculated that excessive cytokine
secretion may activate Th1 cells, and the viral-induced cytokine storm
determines the severity of the disease. Notably, cytokine storms may
directly cause immune cell death, tissue damage, and respiratory
arrest. The study found that the number of immune cells in COVID-19
patients changed, and pathological changes such as spleen atrophy and
necrosis, lymph node necrosis, renal hemorrhage, hepatomegaly, and
degeneration of neurons in the central nervous system were observed.[50]
Studies have also found a correlation between lymphocyte reduction and
liver injury in patients with COVID-19, and increased IL-6 and IL-10
and decreased CD4+ T cells are independent risk factors for severe
liver injury.[45,51]
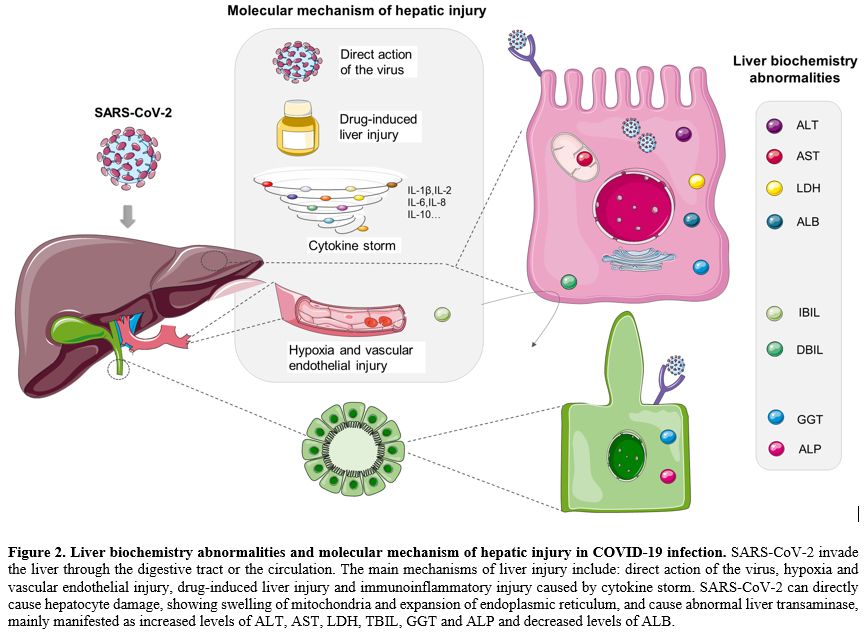 |
Figure 2. Liver biochemistry abnormalities and molecular mechanism of hepatic injury in COVID-19 infection.
SARS-CoV-2 invade the liver through the digestive tract or the
circulation. The main mechanisms of liver injury include: direct action
of the virus, hypoxia and vascular endothelial injury, drug-induced
liver injury and immunoinflammatory injury caused by cytokine storm.
SARS-CoV-2 can directly cause hepatocyte damage, showing swelling of
mitochondria and expansion of endoplasmic reticulum, and cause abnormal
liver transaminase, mainly manifested as increased levels of ALT, AST,
LDH, TBIL, GGT and ALP and decreased levels of ALB. |
Liver Biochemistry Abnormalities in COVID-19 Patients.
Liver function impairment will seriously affect the synthesis and
metabolism of the body. The risk of hepatic injury in COVID-19 patients
during hospitalization was 8.4-58.4%,[52,53] mainly
manifested as increased levels of ALT, AST, GGT, ALP, lactate
dehydrogenase (LDH), and total bilirubin (TBIL) and decreased levels of
albumin (ALB). The changes of liver biochemical indexes in COVID-19
patients mainly reflected liver injury and bile duct injury. We
integrated the liver biochemical indexes of COVID-19 patients in
multiple studies, and their variations are shown in Table 1.
Biochemical Indexes of Liver Injury.
The main indexes of liver injury were ALT, AST, LDH and ALB. Elevated
ALT and/or AST on admission was found in 3.75% to 61.1% of COVID-19
patients. The rate of change during hospital treatment ranged from 8.4%
to 58.4% (Table 1). The
elevation of ALT and AST in most patients was between 1 and 2 times the
upper reference limit, suggesting that mild liver injury was the main
result of COVID-19.[2,54] The
patients with abnormal liver biochemical test results, especially
hepatocytic or mixed type, had a significantly higher risk of
developing severe pneumonia and higher mortality than patients with
normal liver biochemical tests at admission (Table 2).[2,46] An elevated AST/ALT ratio on admission was an independent risk factor for poor prognosis.[55]
Qin et al. found that COVID-19 patients with AST/ALT ≥1.38 had more
severe chest CT findings, poorer laboratory examination results, more
severe disease scores, and worse prognosis.[56] Lei et al. reported a significantly increased mortality risk for patients with AST ranging from >40 U/L.[57]
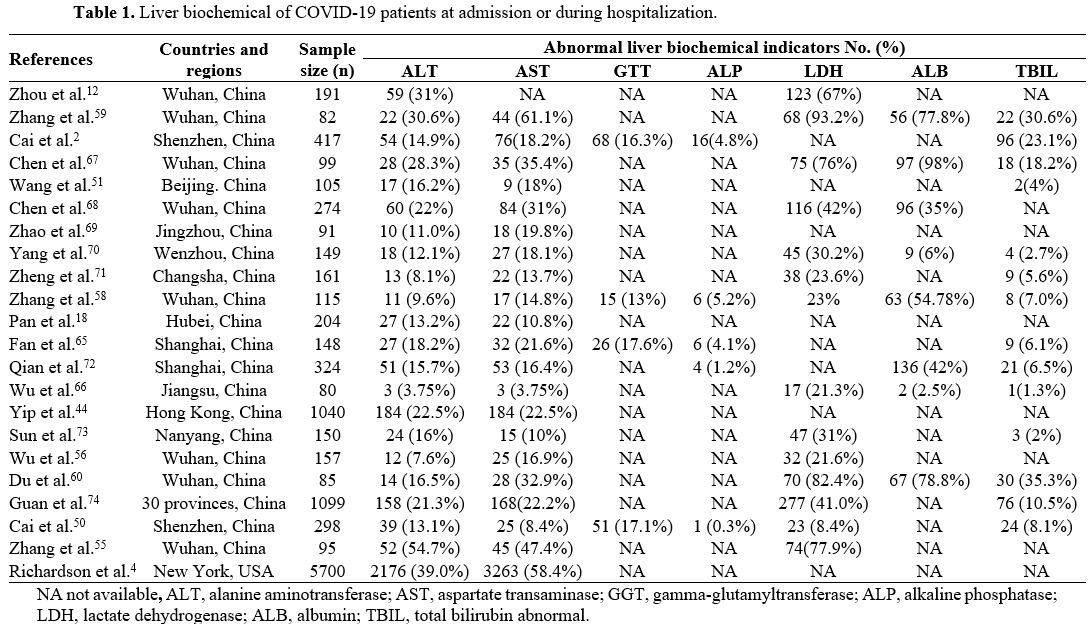 |
Table
1. Liver biochemical of COVID-19 patients at admission or during hospitalization. |
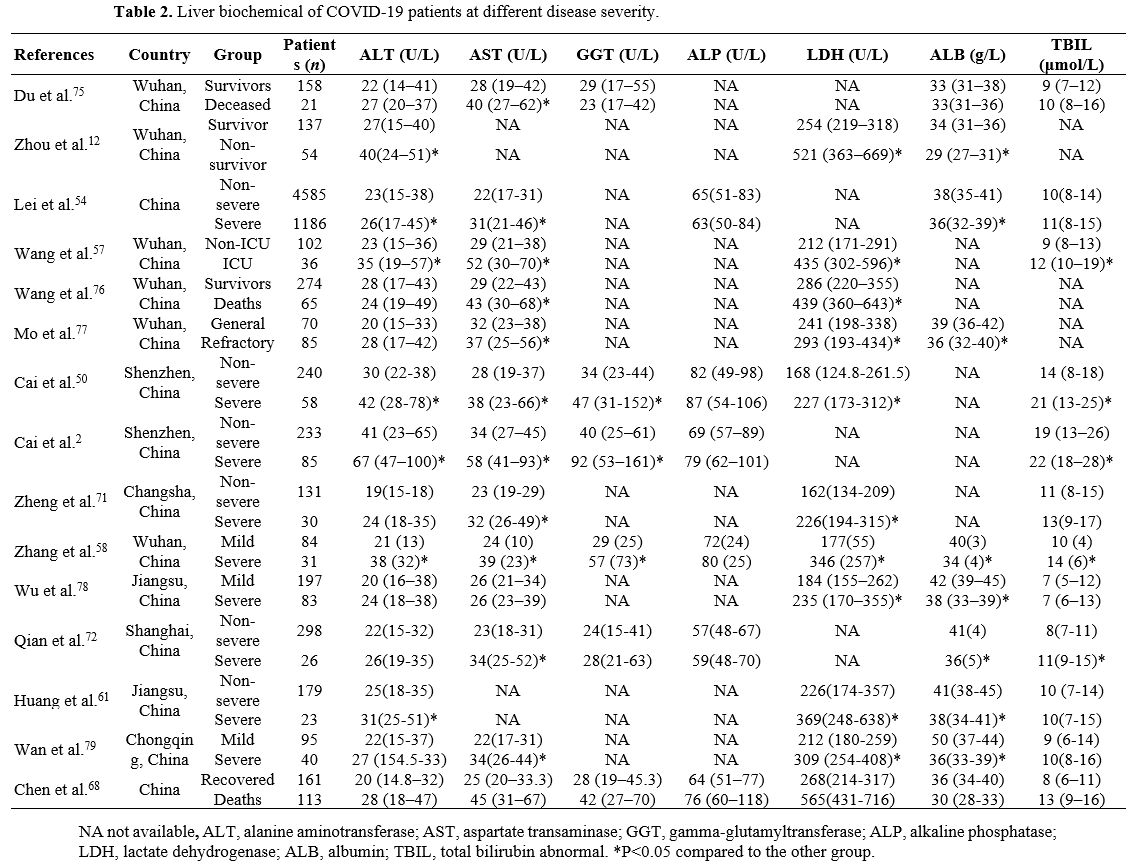 |
Table 2. Liver biochemical of COVID-19 patients at different disease severity. |
LDH levels were significantly increased in COVID-19 patients.[58,59] Patients with severe ICU and refractory COVID-19 had higher LDH levels.[47,60,61] In two retrospective studies of COVID-19 deaths, elevated LDH levels were as high as 82.4% to 93.2%.[62,63]
LDH was positively correlated with COVID-19 severity and was higher in
patients with abnormal liver function than in those with normal liver
function, and it is a good predictor of severe disease.[62,63]
Abnormal
protein metabolism occurs when liver function is impaired, depending on
liver injury type, severity, and duration. Most patients with COVID-19
have decreased albumin after hospitalization, and in some patients, the
decrease is progressive and lower than the pretreatment level (Table 1). The decrease in ALB levels was most pronounced in severe COVID-19 patients.[61,64]
Patients with COVID-19 have gastrointestinal symptoms such as nausea
and vomiting, resulting in reduced nutrient intake and ALB production.
Patients also have a fever, shortness of breath, dyspnea, and diarrhea,
which can lead to increased consumption of ALB. In addition, the
virus-induced release of inflammatory cytokines alters vascular
permeability, leading to extravascular leakage of ALB. Finally,
patients with liver injury have an impaired ability to synthesize ALB,
decreasing ALB levels.
Biochemical Indexes of Bile Duct Injury.
ALP is closely bound to the lipid membrane in liver cells and is not
easily released. While bile acids, by their surface activation, can
dissociate ALP from the lipid membrane, resulting in a significant
increase in serum ALP during cholestasis. Therefore, ALP is a bile duct
cell-related enzyme. The increase in ALP is not obvious in liver injury
but bile duct injury. Generally, ALP is not significantly elevated on
admission in patients with COVID-19, and during the hospitalization,
the rate of elevation of ALP increased to 8.5-11.0%.[46]
Nevertheless,
the elevated rate of ALP was low, and there was no significant
difference in ALP levels between severe COVID-19 patients and milder
COVID-19 patients (Tables 1 and 2).
Like GGT, ALP is considered a bile duct cell-related enzyme, but ALP is
more sensitive to bile duct injury than GGT. Usually, patients with
elevated GGT levels and normal ALP may have drug-induced liver injury.[2,65,66]
Biochemical Indexes of Both Liver and Bile Duct Injury.
GGT and bilirubin were biochemical indexes that could reflect the
injury of the liver and bile duct. GGT is considered as one kind of
"bile duct cell-related enzyme", and its level was significantly
increased in COVID-19 patients.[61,67,68] During hospitalization and in patients with COVID-19 liver injury, GGT increased by 75.6-82.2%.[2,46] Studies have found that GGT levels are significantly higher in severe COVID-19 patients than in mild COVID-19 patients.[61] Although GGT is also considered a bile duct cell-related enzyme, GGT is not sensitive to ALP in bile duct injury.
Direct
bilirubin elevation indicates that the excretion of bilirubin from the
biliary tract after metabolism by liver cells is obstructed. However,
the indirect elevation of bilirubin is usually due to damage to normal
liver cells and disorder of bilirubin metabolism and/or excretion in
the liver, which indicates liver lesions. Abnormal bilirubin in
COVID-19 patients were mainly associated with an increase in indirect
bilirubin.[63,69] Total bilirubin
(TBIL) and direct bilirubin values of COVID-19 patients increased from
the first week compared with baseline levels, suggesting abnormal bile
metabolism in COVID-19 patients in the early stage of the disease.
Studies have found that the levels of TBIL are significantly higher in
severe COVID-19 patients than in mild COVID-19 patients.[47,57]
Conclusions
Liver
injury in COVID-19 patients is common, especially in critically ill
patients. SARS-CoV-2 infected the liver through blood circulation or
retrograde infection of the digestive tract. Liver injury in COVID-19
patients was mainly related to viral infection, hypoxia and endothelial
injury, drug injury and immune injury. Patients generally have
elevations of ALT, AST, GGT, ALP, LDH, bilirubin, and ALB reduction.
Patients with abnormal liver tests are at increased risk of developing
serious disease. In conclusion, more monitoring of liver biochemical
examination in patients with COVID-19 should be carried out, and
liver-protecting therapy should be given based on the active treatment
of the primary disease to reduce liver injury.
Abbreviation
COVID-19,
coronavirus disease 2019; SARS-CoV2, severe acute respiratory
syndrome coronavirus 2; ALT, alanine aminotransferase; AST, aspartate
aminotransferase; ALP, alkaline phosphatase; GGT,
gamma-glutamyltransferase; LDH, lactate dehydrogenase; TBIL, total
bilirubin; ALB,albumin; ACE, angiotensin-converting enzyme; ICU,
intensive care unit.
Acknowledgements
We thank the authors of the primary studies for their timely and helpful responses to our information requests.
Funding
Supported
by Health and Technology Foundation of Jilin Province-Youth Science
Fund, No. 2020Q009. Natural Science Foundation of Jilin Province,
No.20200201568JC, Special project of medical and health talents in
Jilin Province, No. 2019SRCJ021, Science and technology research
project of Jilin Provincial Department of Education, No.
JJKH20211151KJ, Cultivation project of National Natural Science
Foundation of the second hospital of Jilin University, No. KYPY2018-21.
References
- Cascella M, Rajnik M,
Aleem A, Dulebohn SC, Di
Napoli R. Features, Evaluation, and Treatment of Coronavirus
(COVID-19). StatPearls. Treasure Island (FL)2021.
- Cai Q, Huang D, Yu H, et
al. COVID-19: Abnormal liver function tests. J Hepatol. Sep
2020;73(3):566-574. https://doi.org/10.1016/j.jhep.2020.04.006
PMid:32298767 PMCid:PMC7194951
- Wu
C, Chen X, Cai Y, et al. Risk Factors Associated With Acute Respiratory
Distress Syndrome and Death in Patients With Coronavirus Disease 2019
Pneumonia in Wuhan, China. JAMA Intern Med. Jul 1 2020;180(7):934-943. https://doi.org/10.1001/jamainternmed.2020.0994
PMid:32167524 PMCid:PMC7070509
- Wang
Y, Liu S, Liu H, et al. SARS-CoV-2 infection of the liver directly
contributes to hepatic impairment in patients with COVID-19. J Hepatol.
Oct 2020;73(4):807-816. https://doi.org/10.1016/j.jhep.2020.05.002
PMid:32437830 PMCid:PMC7211738
- Zhao
Y, Zhao Z, Wang Y, Zhou Y, Ma Y, Zuo W. Single-Cell RNA Expression
Profiling of ACE2, the Receptor of SARS-CoV-2. Am J Respir Crit Care
Med. Sep 1 2020;202(5):756-759. https://doi.org/10.1164/rccm.202001-0179LE
PMid:32663409 PMCid:PMC7462411
- Wrapp
D, Wang N, Corbett KS, et al. Cryo-EM structure of the 2019-nCoV spike
in the prefusion conformation. Science. Mar 13
2020;367(6483):1260-1263. https://doi.org/10.1126/science.abb2507
PMid:32075877 PMCid:PMC7164637
- Hikmet
F, Mear L, Edvinsson A, Micke P, Uhlen M, Lindskog C. The protein
expression profile of ACE2 in human tissues. Mol Syst Biol. Jul
2020;16(7):e9610. https://doi.org/10.15252/msb.20209610
PMid:32715618 PMCid:PMC7383091
- Harmer
D, Gilbert M, Borman R, Clark KL. Quantitative mRNA expression
profiling of ACE 2, a novel homologue of angiotensin-converting enzyme.
FEBS Lett. Dec 4 2002;532(1-2):107-110. https://doi.org/10.1016/S0014-5793(02)03640-2
- Uhlen
M, Fagerberg L, Hallstrom BM, et al. Proteomics. Tissue-based map of
the human proteome. Science. Jan 23 2015;347(6220):1260419.
- Hamming
I, Timens W, Bulthuis ML, Lely AT, Navis G, van Goor H. Tissue
distribution of ACE2 protein, the functional receptor for SARS
coronavirus. A first step in understanding SARS pathogenesis. J Pathol.
Jun 2004;203(2):631-637. https://doi.org/10.1002/path.1570
PMid:15141377 PMCid:PMC7167720
- Yao
XH, Li TY, He ZC, et al. [A pathological report of three COVID-19 cases
by minimal invasive autopsies]. Zhonghua Bing Li Xue Za Zhi. May 8
2020;49(5):411-417.
- Zhou F, Yu T, Du
R, et al. Clinical course and risk factors for mortality of adult
inpatients with COVID-19 in Wuhan, China: a retrospective cohort study.
Lancet. Mar 28 2020;395(10229):1054-1062. https://doi.org/10.1016/S0140-6736(20)30566-3
- Sonzogni
A, Previtali G, Seghezzi M, et al. Liver histopathology in severe COVID
19 respiratory failure is suggestive of vascular alterations. Liver
Int. Sep 2020;40(9):2110-2116. https://doi.org/10.1111/liv.14601
PMid:32654359 PMCid:PMC7404964
- Guo
M, Tao W, Flavell RA, Zhu S. Potential intestinal infection and
faecal-oral transmission of SARS-CoV-2. Nat Rev Gastroenterol Hepatol.
Apr 2021;18(4):269-283. https://doi.org/10.1038/s41575-021-00416-6
PMid:33589829 PMCid:PMC7883337
- Lamers
MM, Beumer J, van der Vaart J, et al. SARS-CoV-2 productively infects
human gut enterocytes. Science. Jul 3 2020;369(6499):50-54. https://doi.org/10.1126/science.abc1669
PMid:32358202 PMCid:PMC7199907
- Qian Q, Fan L, Liu W,
et al. Direct evidence of active SARS-CoV-2 replication in the
intestine. Clin Infect Dis. Jul 8 2020. https://doi.org/10.1093/cid/ciaa925
PMid:32638022 PMCid:PMC7454471
- Ng SC, Tilg H. COVID-19
and the gastrointestinal tract: more than meets the eye. Gut. Jun
2020;69(6):973-974. https://doi.org/10.1136/gutjnl-2020-321195
PMid:32273292 PMCid:PMC7211058
- Pan
L, Mu M, Yang P, et al. Clinical Characteristics of COVID-19 Patients
With Digestive Symptoms in Hubei, China: A Descriptive,
Cross-Sectional, Multicenter Study. Am J Gastroenterol. May
2020;115(5):766-773. https://doi.org/10.14309/ajg.0000000000000620
PMid:32287140 PMCid:PMC7172492
- Assante
G, Williams R, Youngson NA. Is the increased risk for MAFLD patients to
develop severe COVID-19 linked to perturbation of the gut-liver axis? J
Hepatol. Feb 2021;74(2):487-488. https://doi.org/10.1016/j.jhep.2020.05.051
PMid:32574578 PMCid:PMC7305888
- Albillos
A, de Gottardi A, Rescigno M. The gut-liver axis in liver disease:
Pathophysiological basis for therapy. J Hepatol. Mar
2020;72(3):558-577. https://doi.org/10.1016/j.jhep.2019.10.003
PMid:31622696
- Cheung
OY, Chan JW, Ng CK, Koo CK. The spectrum of pathological changes in
severe acute respiratory syndrome (SARS). Histopathology. Aug
2004;45(2):119-124. https://doi.org/10.1111/j.1365-2559.2004.01926.x
PMid:15279629 PMCid:PMC7194176
- Chen J, Subbarao K. The
Immunobiology of SARS*. Annu Rev Immunol. 2007;25:443-472. https://doi.org/10.1146/annurev.immunol.25.022106.141706
PMid:17243893
- Puelles
VG, Lutgehetmann M, Lindenmeyer MT, et al. Multiorgan and Renal Tropism
of SARS-CoV-2. N Engl J Med. Aug 6 2020;383(6):590-592. https://doi.org/10.1056/NEJMc2011400
PMid:32402155 PMCid:PMC7240771
- Zhang
C, Shi L, Wang FS. Liver injury in COVID-19: management and challenges.
Lancet Gastroenterol Hepatol. May 2020;5(5):428-430. https://doi.org/10.1016/S2468-1253(20)30057-1
- Jothimani D, Venugopal
R, Abedin MF, Kaliamoorthy I, Rela M. COVID-19 and the liver. J
Hepatol. Nov 2020;73(5):1231-1240. https://doi.org/10.1016/j.jhep.2020.06.006
PMid:32553666 PMCid:PMC7295524
- Zhao
B, Ni C, Gao R, et al. Recapitulation of SARS-CoV-2 infection and
cholangiocyte damage with human liver ductal organoids. Protein Cell.
Oct 2020;11(10):771-775. https://doi.org/10.1007/s13238-020-00718-6
PMid:32303993 PMCid:PMC7164704
- Zhong
P, Xu J, Yang D, et al. COVID-19-associated gastrointestinal and liver
injury: clinical features and potential mechanisms. Signal Transduct
Target Ther. Nov 2 2020;5(1):256. https://doi.org/10.1038/s41392-020-00373-7
PMid:33139693 PMCid:PMC7605138
- Paizis
G, Tikellis C, Cooper ME, et al. Chronic liver injury in rats and
humans upregulates the novel enzyme angiotensin converting enzyme 2.
Gut. Dec 2005;54(12):1790-1796. https://doi.org/10.1136/gut.2004.062398
PMid:16166274 PMCid:PMC1774784
- Nardo
AD, Schneeweiss-Gleixner M, Bakail M, Dixon ED, Lax SF, Trauner M.
Pathophysiological mechanisms of liver injury in COVID-19. Liver Int.
Jan 2021;41(1):20-32. https://doi.org/10.1111/liv.14730
PMid:33190346 PMCid:PMC7753756
- Suarez-Farinas
M, Tokuyama M, Wei G, et al. Intestinal Inflammation Modulates the
Expression of ACE2 and TMPRSS2 and Potentially Overlaps With the
Pathogenesis of SARS-CoV-2-related Disease. Gastroenterology. Jan
2021;160(1):287-301 e220. https://doi.org/10.1053/j.gastro.2020.09.029
PMid:32980345 PMCid:PMC7516468
- Gkogkou
E, Barnasas G, Vougas K, Trougakos IP. Expression profiling
meta-analysis of ACE2 and TMPRSS2, the putative anti-inflammatory
receptor and priming protease of SARS-CoV-2 in human cells, and
identification of putative modulators. Redox Biol. Sep 2020;36:101615. https://doi.org/10.1016/j.redox.2020.101615
PMid:32863223 PMCid:PMC7311357
- Varga
Z, Flammer AJ, Steiger P, et al. Endothelial cell infection and
endotheliitis in COVID-19. Lancet. May 2 2020;395(10234):1417-1418. https://doi.org/10.1016/S0140-6736(20)30937-5
- Ackermann
M, Verleden SE, Kuehnel M, et al. Pulmonary Vascular Endothelialitis,
Thrombosis, and Angiogenesis in Covid-19. N Engl J Med. Jul 9
2020;383(2):120-128. https://doi.org/10.1056/NEJMoa2015432
PMid:32437596 PMCid:PMC7412750
- Codo
AC, Davanzo GG, Monteiro LB, et al. Elevated Glucose Levels Favor
SARS-CoV-2 Infection and Monocyte Response through a
HIF-1alpha/Glycolysis-Dependent Axis. Cell Metab. Sep 1
2020;32(3):437-446 e435. https://doi.org/10.2139/ssrn.3606770
- Erlich
JR, To EE, Liong S, et al. Targeting Evolutionary Conserved Oxidative
Stress and Immunometabolic Pathways for the Treatment of Respiratory
Infectious Diseases. Antioxid Redox Signal. May 1 2020;32(13):993-1013.
https://doi.org/10.1089/ars.2020.8028
PMid:32008371 PMCid:PMC7426980
- Fontana
RJ. Pathogenesis of idiosyncratic drug-induced liver injury and
clinical perspectives. Gastroenterology. Apr 2014;146(4):914-928. https://doi.org/10.1053/j.gastro.2013.12.032
PMid:24389305 PMCid:PMC4031195
- Wang
Y, Lin Z, Liu Z, et al. A unifying ontology to integrate histological
and clinical observations for drug-induced liver injury. Am J Pathol.
Apr 2013;182(4):1180-1187. https://doi.org/10.1016/j.ajpath.2012.12.033
PMid:23395088
- Olry
A, Meunier L, Delire B, Larrey D, Horsmans Y, Le Louet H. Drug-Induced
Liver Injury and COVID-19 Infection: The Rules Remain the Same. Drug
Saf. Jul 2020;43(7):615-617. https://doi.org/10.1007/s40264-020-00954-z
PMid:32514859 PMCid:PMC7279629
- Lan
NT, Thu NT, Barrail-Tran A, et al. Randomised pharmacokinetic trial of
rifabutin with lopinavir/ritonavir-antiretroviral therapy in patients
with HIV-associated tuberculosis in Vietnam. PLoS One.
2014;9(1):e84866. https://doi.org/10.1371/journal.pone.0084866
PMid:24465443 PMCid:PMC3898920
- Falcao
MB, Pamplona de Goes Cavalcanti L, Filgueiras Filho NM, Antunes de
Brito CA. Case Report: Hepatotoxicity Associated with the Use of
Hydroxychloroquine in a Patient with COVID-19. Am J Trop Med Hyg. Jun
2020;102(6):1214-1216. https://doi.org/10.4269/ajtmh.20-0276
PMid:32314698 PMCid:PMC7253107
- Martinez
MA, Vuppalanchi R, Fontana RJ, et al. Clinical and histologic features
of azithromycin-induced liver injury. Clin Gastroenterol Hepatol. Feb
2015;13(2):369-376 e363.
https://doi.org/10.1016/j.cgh.2014.07.054
PMid:25111234 PMCid:PMC4321982
- Muhovic
D, Bojovic J, Bulatovic A, et al. First case of drug-induced liver
injury associated with the use of tocilizumab in a patient with
COVID-19. Liver Int. Aug 2020;40(8):1901-1905. https://doi.org/10.1111/liv.14516
PMid:32478465 PMCid:PMC7276916
- Pavlov
CS, Varganova DL, Casazza G, Tsochatzis E, Nikolova D, Gluud C.
Glucocorticosteroids for people with alcoholic hepatitis. Cochrane
Database Syst Rev. Nov 2 2017;11:CD001511. https://doi.org/10.1002/14651858.CD001511.pub3
PMid:29096421 PMCid:PMC6491283
- Ferron
PJ, Gicquel T, Megarbane B, Clement B, Fromenty B. Treatments in
Covid-19 patients with pre-existing metabolic dysfunction-associated
fatty liver disease: A potential threat for drug-induced liver injury?
Biochimie. Dec 2020;179:266-274. https://doi.org/10.1016/j.biochi.2020.08.018
PMid:32891697 PMCid:PMC7468536
- Zhan
K, Liao S, Li J, et al. Risk factors in patients with COVID-19
developing severe liver injury during hospitalization. Gut. Mar
2021;70(3):628-629. https://doi.org/10.1136/gutjnl-2020-321913
PMid:32571973 PMCid:PMC7873415
- Yip
TC, Lui GC, Wong VW, et al. Liver injury is independently associated
with adverse clinical outcomes in patients with COVID-19. Gut. Jul 8
2020. https://doi.org/10.1136/gutjnl-2020-321726
PMid:32641471 PMCid:PMC7371491
- Huang
C, Wang Y, Li X, et al. Clinical features of patients infected with
2019 novel coronavirus in Wuhan, China. Lancet. Feb 15
2020;395(10223):497-506. https://doi.org/10.1016/S0140-6736(20)30183-5
- Nile
SH, Nile A, Qiu J, Li L, Jia X, Kai G. COVID-19: Pathogenesis, cytokine
storm and therapeutic potential of interferons. Cytokine Growth Factor
Rev. Jun 2020;53:66-70. https://doi.org/10.1016/j.cytogfr.2020.05.002
PMid:32418715 PMCid:PMC7204669
- Yalcin
AD, Yalcin AN. Future perspective: biologic agents in patients with
severe COVID-19. Immunopharmacol Immunotoxicol. Feb 2021;43(1):1-7. https://doi.org/10.1080/08923973.2020.1818770
PMid:32883116
- Park MD. Macrophages: a
Trojan horse in COVID-19? Nat Rev Immunol. Jun 2020;20(6):351. https://doi.org/10.1038/s41577-020-0317-2
PMid:32303696 PMCid:PMC7186930
- Han
H, Ma Q, Li C, et al. Profiling serum cytokines in COVID-19 patients
reveals IL-6 and IL-10 are disease severity predictors. Emerg Microbes
Infect. Dec 2020;9(1):1123-1130. https://doi.org/10.1080/22221751.2020.1770129
PMid:32475230 PMCid:PMC7473317
- Richardson
S, Hirsch JS, Narasimhan M, et al. Presenting Characteristics,
Comorbidities, and Outcomes Among 5700 Patients Hospitalized With
COVID-19 in the New York City Area. JAMA. May 26
2020;323(20):2052-2059.
- Cai Q, Huang
D, Ou P, et al. COVID-19 in a designated infectious diseases hospital
outside Hubei Province, China. Allergy. Jul 2020;75(7):1742-1752. https://doi.org/10.1111/all.14309
PMid:32239761
- Wang
Q, Zhao H, Liu LG, et al. Pattern of liver injury in adult patients
with COVID-19: a retrospective analysis of 105 patients. Mil Med Res.
Jun 7 2020;7(1):28. https://doi.org/10.21203/rs.3.rs-20849/v2
- Fu
T, Deng M, Wang X, Yang F. Predictor of poor prognosis of COVID-19
patients - liver injury. Expert Rev Gastroenterol Hepatol. Oct
2020;14(10):873-876. https://doi.org/10.1080/17474124.2020.1793670
PMid:32643980
- Qin
C, Wei Y, Lyu X, et al. High aspartate aminotransferase to alanine
aminotransferase ratio on admission as risk factor for poor prognosis
in COVID-19 patients. Sci Rep. Oct 5 2020;10(1):16496. https://doi.org/10.1038/s41598-020-73575-2
PMid:33020546 PMCid:PMC7536227
- Lei
F, Liu YM, Zhou F, et al. Longitudinal Association Between Markers of
Liver Injury and Mortality in COVID-19 in China. Hepatology. Aug
2020;72(2):389-398. https://doi.org/10.1002/hep.31301
PMid:32359177 PMCid:PMC7267515
- Zhang
G, Zhang J, Wang B, Zhu X, Wang Q, Qiu S. Analysis of clinical
characteristics and laboratory findings of 95 cases of 2019 novel
coronavirus pneumonia in Wuhan, China: a retrospective analysis.
Respiratory Research. Mar 26 2020;21(1). https://doi.org/10.1186/s12931-020-01338-8
PMid:32216803 PMCid:PMC7099829
- Wu
H, Zhu H, Yuan C, et al. Clinical and Immune Features of Hospitalized
Pediatric Patients With Coronavirus Disease 2019 (COVID-19) in Wuhan,
China. JAMA Netw Open. Jun 1 2020;3(6):e2010895. https://doi.org/10.1001/jamanetworkopen.2020.10895
PMid:32492165 PMCid:PMC7272117
- Wang
D, Hu B, Hu C, et al. Clinical Characteristics of 138 Hospitalized
Patients With 2019 Novel Coronavirus-Infected Pneumonia in Wuhan,
China. JAMA. Mar 17 2020;323(11):1061-1069. https://doi.org/10.1001/jama.2020.1585
PMid:32031570 PMCid:PMC7042881
- Zhang
Y, Zheng L, Liu L, Zhao M, Xiao J, Zhao Q. Liver impairment in COVID-19
patients: A retrospective analysis of 115 cases from a single centre in
Wuhan city, China. Liver International. Sep 2020;40(9):2095-2103. https://doi.org/10.1111/liv.14455
PMid:32239796
- Zhang B, Zhou X, Qiu Y,
et al. Clinical characteristics of 82 cases of death from COVID-19.
PLoS One. 2020;15(7):e0235458. https://doi.org/10.1371/journal.pone.0235458
PMid:32645044 PMCid:PMC7347130
- Du
Y, Tu L, Zhu P, et al. Clinical Features of 85 Fatal Cases of COVID-19
from Wuhan. A Retrospective Observational Study. Am J Respir Crit Care
Med. Jun 1 2020;201(11):1372-1379. https://doi.org/10.1164/rccm.202003-0543OC
PMid:32242738 PMCid:PMC7258652
- Huang
R, Zhu L, Xue L, et al. Clinical findings of patients with coronavirus
disease 2019 in Jiangsu province, China: A retrospective, multi-center
study. PLoS Negl Trop Dis. May 2020;14(5):e0008280. https://doi.org/10.1371/journal.pntd.0008280
PMid:32384078 PMCid:PMC7239492
- Dillon
JF, Miller MH. Gamma glutamyl transferase 'To be or not to be' a liver
function test? Ann Clin Biochem. Nov 2016;53(6):629-631. https://doi.org/10.1177/0004563216659887
PMid:27384446
- Fernandez NJ, Kidney
BA. Alkaline phosphatase: beyond the liver. Vet Clin Pathol. Sep
2007;36(3):223-233. https://doi.org/10.1111/j.1939-165X.2007.tb00216.x
PMid:17806069
- Zhao
D, Yao F, Wang L, et al. A Comparative Study on the Clinical Features
of Coronavirus 2019 (COVID-19) Pneumonia With Other Pneumonias. Clin
Infect Dis. Jul 28 2020;71(15):756-761. https://doi.org/10.1093/cid/ciaa247
PMid:32161968 PMCid:PMC7108162
- Fan
Z, Chen L, Li J, et al. Clinical Features of COVID-19-Related Liver
Functional Abnormality. Clin Gastroenterol Hepatol. Jun
2020;18(7):1561-1566. https://doi.org/10.1016/j.cgh.2020.04.002
PMid:32283325 PMCid:PMC7194865
- Wu
J, Liu J, Zhao X, et al. Clinical Characteristics of Imported Cases of
Coronavirus Disease 2019 (COVID-19) in Jiangsu Province: A Multicenter
Descriptive Study. Clin Infect Dis. Jul 28 2020;71(15):706-712. https://doi.org/10.1093/cid/ciaa199
PMid:32109279 PMCid:PMC7108195
[TOP]