Hong Li, Kehong Bi, Saran Feng, Yan Wang and Chuansheng Zhu.
Department of
Hematology, The First Affiliated Hospital of Shandong First Medical
University & Shandong Provincial Qianfoshan Hospital, No. 16766
Jingshi Road, Jinan 250014, Shandong Province, P.R. China.
Correspondence to:
Hong Li, Department of Hematology, The First Affiliated Hospital of
Shandong First Medical University & Shandong Provincial Qianfoshan
Hospital, No. 16766 Jingshi Road, Jinan 250014, Shandong Province, P.R.
China
Published: January 1, 2022
Received: September 9, 2021
Accepted: December 6, 2021
Mediterr J Hematol Infect Dis 2022, 14(1): e2022005 DOI
10.4084/MJHID.2022.005
This is an Open Access article distributed
under the terms of the Creative Commons Attribution License
(https://creativecommons.org/licenses/by-nc/4.0),
which permits unrestricted use, distribution, and reproduction in any
medium, provided the original work is properly cited.
|
Abstract
Objectives:
MiR-140 and DNAJC3-AS1 have been demonstrated to play critical roles in
cancer biology, while their participation in acute myeloid leukemia
(AML) is unclear. This study aimed to explore the role of miR-140 and
DNAJC3-AS1 in AML. Methods:
The expression of DNAJC3-AS1 and miR-140 were detected by RT-qPCR.
Then, the role of DNAJC3-AS1 and miR-140 in regulating each other was
explored by overexpression assay. Next, the direct interaction between
DNAJC3-AS1 and miR-140 was analyzed using an RNA pull-down assay. Next,
the subcellular location of DNAJC3-AS1 was explored using cellular,
subcellular fractionation assay. Finally, cell proliferation analysis
was evaluated with BrdU assay. Results:
Increased expression levels of DNAJC3-AS1 and decreased expression
levels of miR-140 were observed in AML patients. DNAJC3-AS1 was
detected in nuclear and cytoplasm samples and direct interaction
between DNAJC3-AS1 and miR-140 was observed. Discussion:
Reduced expression levels of DNAJC3-AS1 were observed after
overexpression of miR-140 in AML cells. DNAJC3-AS1 increased cell
proliferation and inhibited the role of miR-140 in suppressing cell
proliferation. Conclusion: In conclusion, miR-140 may target DNAJC3-AS1 to suppress cell proliferation in AML.
|
Introduction
As
the most common type of myeloid leukemia, acute myeloid leukemia (AML)
develops from bone marrow, where blood cells are produced.[1]
With disease development, AML cells will move into the blood quickly.
They may even move to lymph nodes and many important organs of the
human body, such as the spleen, liver, testicles, and even the central
nervous system, leading to poor prognosis.[2,3] AML is estimated to affect about 4.3 out of 100,000 people each year.[3] Moreover, only less than 28% of these patients could survive more than five years after the initial diagnosis.[4,5] AML patients are frequently treated with chemotherapy,[6,7] while AML mainly affects patients older than 65 years and usually cannot tolerate the adverse effects of chemotherapy.[6,7] Therefore, treatment strategies of AML should be improved.
Targeted
therapies have been proven to be potential novel targets for the
treatment of AML because of the high specificity and fewer adverse
effects.[8,9] Some signal pathways, such as the hedgehog pathway and the PI3K/AKT pathway, have shown promising potentials to treat AML.[10,11]
However, more effective targets with higher safety are needed to
improve targeted therapies for AML further. Noncoding RNA (ncRNAs),
such as microRNAs (miRNAs) and long ncRNAs (lncRNAs), lack
protein-coding capacity but affect protein synthesis to regulate cancer
progression.[12,13] Therefore, ncRNAs are potential
candidates for AML treatment. MiR-140 and DNAJC3-AS1 have been proven
to play critical roles in cancer biology, while their participation in
AML is unclear.[14-17] Therefore, we predicted that
miR-140 might target DNAJC3-AS1. Therefore, this study was conducted to
explore the interaction between DNAJC3-AS1 and miR-140 in AML.
Materials and Methods
Patients and Samples of Peripheral Blood Mononuclear Cells (PBMCs).
Blood samples were donated by a total of 49 AML patients (30 males and
19 females, 66.7 +/- 5.9 years old) and 49 healthy controls (30 males
and 19 females, 66.8 +/- 5.6 years old) at the First Affiliated
Hospital of Shandong First Medical University & Shandong Provincial
Qianfoshan Hospital from May 2018 to May 2020. Blood was extracted from
patients after overnight fasting. FicolHistopaque gradient
centrifugation method was used to separate PBMCs. All healthy controls
exhibited normal physiological parameters during a systemic
physiological examination. All 49 AML patients were classified
following the French-American-British (FAB) classification, and 12, 11,
10, and 16 participants were classified into M1/M2, M3, M4/5, and M6/7,
respectively. No anti-AML therapy was performed on these patients
before admission. This study was approved by the Ethics Committee of
the hospital mentioned above. All patients and controls signed the
informed consent.
AML Cells and Cell Culture.
Two AML cell lines HL60 and U937, were purchased from BeNa Culture
Collection (Suzhou, China). DMEM medium (Gibco) was supplemented with
10% FBS (Gibco), 100 U/ml penicillin, and 100 μg/ml streptomycin
(Invitrogen) to serve as the culture medium of both cell lines. Cells
were cultivated at 37 °C with 5% CO2 and 95% humidity.
Cell Transfections.
DNAJC3-AS1 and miR-140 were overexpressed in HL60, and U937 cells by
transfecting DNAJC3-AS1 vector (pcDNA3.1 backbone) and/or miR-140 mimic
(RiboBio, China). All transfections were mediated by Neon
Electroporation Transfection device (Thermo Fisher Scientific).
Negative control (NC) experiments were performed, transfecting cells
with empty vector or NC miRNA. Untransfected cells were cultivated
until the following assays to serve as the control. The overexpression
was checked every 24 h until 96 h.
Preparation of RNA Samples.
EZ RNA Miniprep Kit (EZBioResearch) was used to prepare RNA samples
from PBMCs and HL60 and U937 cells. RNA samples were digested with
DNase I (Invitrogen, USA) to remove genomic DNA. The integrity and RNA
concentrations were evaluated by Bioanalyzer. A RIN value higher than
8.0 was reached in all cases, indicating high RNA integrity.
RT-qPCRs.
With 3,000 ng total RNAs as the template, cDNA samples were prepared
through reverse transcriptions (RTs). With cDNA samples as the
template, the expression levels of DNAJC3-AS1 and miR-140 were
determined by performing qPCRs. The endogenous control for DNAJC3-AS1
and miR-140 was 18S rRNA and U6, respectively. Ct values of DNAJC3-AS1
and miR-140 were normalized to their endogenous control using the 2–ΔΔCt method.
Subcellular Fractionation Assay.
Cytoplasmic and nuclear samples were separated from both HL60 and U937
cells using the NE-PER Nuclear and Cytoplasmic Extraction kit (Thermo
Fisher Scientific). The two fractions were separated by centrifuging
cell lysate at 1,200 g for 10 min. Then, the cytoplasmic sample was
added into an RNase-free tube, while a nuclear sample was incubated
with nuclear lysis buffer on ice for another 60 min. RNA isolation from
these two samples was performed, and RT-PCRs were followed to determine
the expression levels of DNAJC3-AS1. PCR products were separated by 2%
agarose gel. After staining with EB, images were taken with a MyECL
imager.
RNA Pull-Down Assay.
DNAJC3-AS1 or NC RNA expression vector was linearized using T7 RNA
polymerase (Thermo Fisher Scientific) to prepare a biotinylated in
vitro transcript of both DNAJC3-AS1 and NC RNA. The biotinylated RNAs
were named Bio-DNAJC3-AS1 or Bio-NC. These two RNAs were transfected
into HL60 and U937 cells, followed by cell lysis for 48 h.
Streptavidin-agarose beads were then used to pull down the complex,
followed by RNA isolation and RT-qPCRs to determine the expression
levels of miR-140.
BrdU Cell Proliferation Assay.
In this assay, the incorporation of 5-Bromo-2-deoxyUridine (BrdU),
which reflects DNA synthesis, was determined to quantify cell
proliferation. Transfected cells were incubated in a medium containing
10 µM BrdU (BD Pharmingen) for 48 h, then incubated with
anti-BrdU-antibody (peroxidase-coupled, Sigma–Aldrich) at room
temperature for 60 min. After three washing times with PBS, the cells
were incubated with peroxidase substrate for 30 minutes. Finally, OD
values at 450 nm were determined to reflect cell proliferation.
Statistical Analysis.
The unpaired t-test and ANOVA Tukey’s test were used to compare two or
multiple independent groups. Correlation analysis was performed with
Pearson’s correlation coefficient. P < 0.05 was statistically significant.
Results
The Expression of DNAJC3-AS1 and miR-140 in AML Patients and Controls.
Samples of PBMCs were subjected to RT-qPCRs to detect the expression of
DNAJC3-AS1 and miR-140 in AML patients and controls. The results showed
increased expression levels of DNAJC3-AS1 (Figure 1A, p < 0.01) and decreased expression levels of miR-140 (Figure 1B, p
< 0.01) in AML patients. Our data illustrated the potential
participation of these two ncRNAs in AML. Correlation analysis
performed with Pearson’s correlation coefficient revealed that
DNAJC3-AS1 and miR-140 were inversely correlated across both AML (Figure 1C) and control (Figure 1D) samples, suggesting the potential interaction between them.
To
detect the expression of DNAJC3-AS1 (A) and miR-140 (B) in AML patients
and controls, samples of PBMCs were subjected to RNA isolations,
followed by RT-qPCRs. Pearson’s correlation coefficient was applied to
explore the correlations between DNAJC3-AS1 and miR-140 across AML and
control samples. **, p < 0.01.
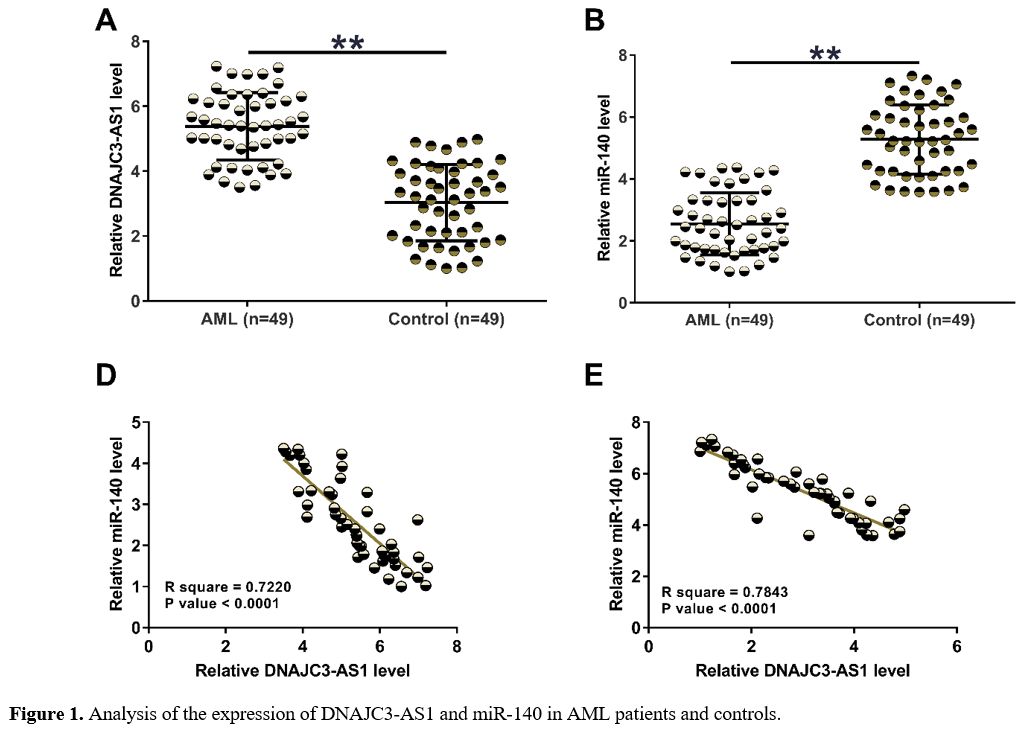 |
Figure 1. Analysis of the expression of DNAJC3-AS1 and miR-140 in AML patients and controls. |
The Direct Interaction Between DNAJC3-AS1 and miR-140 and the Subcellular Location of DNAJC3-AS1.
The online program IntaRNA 2.0 was applied to explore the potential
interaction between DNAJC3-AS1 and miR-140. It was observed that
DNAJC3-AS1 and miR-140 could form potential base pairing (Figure 2A).
RNA-pull down assay was performed to validate the direct interaction
between DNAJC3-AS1 and miR-140 in both HL60 and U937 cells. Compared to
the Bio-DNAJC3-AS1 pull-down group, the Bio-NC pull-down group
exhibited significantly lower expression levels of miR-140, confirming
the direct interaction between DNAJC3-AS1 and miR-140 (Figure 2B, p
< 0.01). Subcellular location of DNAJC3-AS1 in both cell lines was
explored with a subcellular fractionation assay. Unlike GAPDH, the
expression of DNAJC3-AS1 was detected in both nuclear and cytoplasm
samples (Figure 2C).
The
online program IntaRNA 2.0 was applied to explore the potential
interaction between DNAJC3-AS1 and miR-140 (A). RNA-pull down assay was
performed to validate the direct interaction between DNAJC3-AS1 and
miR-140 in both HL60 and U937 cells (B). Subcellular location of
DNAJC3-AS1 in both cell lines was explored with subcellular
fractionation assay (C). **, p < 0.01.
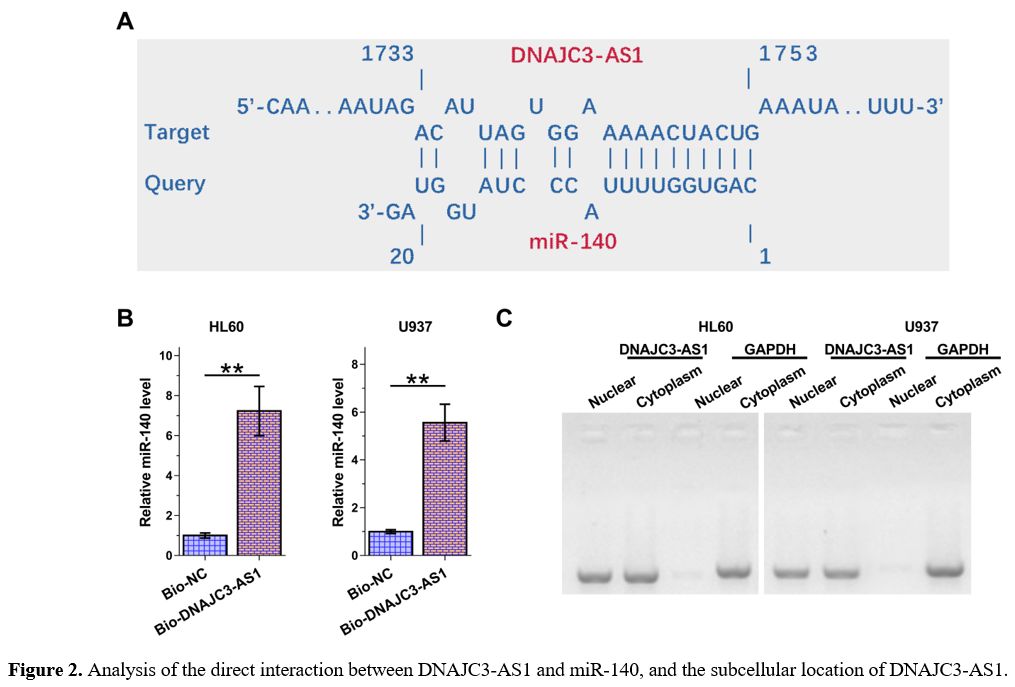 |
Figure 2. Analysis of the direct interaction between DNAJC3-AS1 and miR-140, and the subcellular location of DNAJC3-AS1. |
The Role of miR-140 in the Expression of DNAJC3-AS1.
DNAJC3-AS1 or miR-140 was overexpressed in HL60 and U937 cells,
followed by confirmation of the expression of DNAJC3-AS1 and miR-140
every 24 h until 96 h (Figure 3A, p < 0.05). Reduced expression levels of DNAJC3-AS1 were observed after the overexpression of miR-140 in both cells (Figure 3B, p < 0.05). In contrast, no altered expression of miR-140 was observed after the overexpression of DNAJC3-AS1 (Figure 3C). Therefore, DNAJC3-AS1 could be targeted by miR-140 in AML cells.
HL60
and U937 cells were overexpressed with DNAJC3-AS1 or miR-140, followed
by confirming the expression of DNAJC3-AS1 and miR-140 every 24 h until
96 h (A). The role of miR-140 in the expression of DNAJC3-AS1 (B) and
the role of DNAJC3-AS1 in the expression of miR-140 (C) were explored
by overexpression assays, followed by RT-qPCRs. *, p < 0.01.
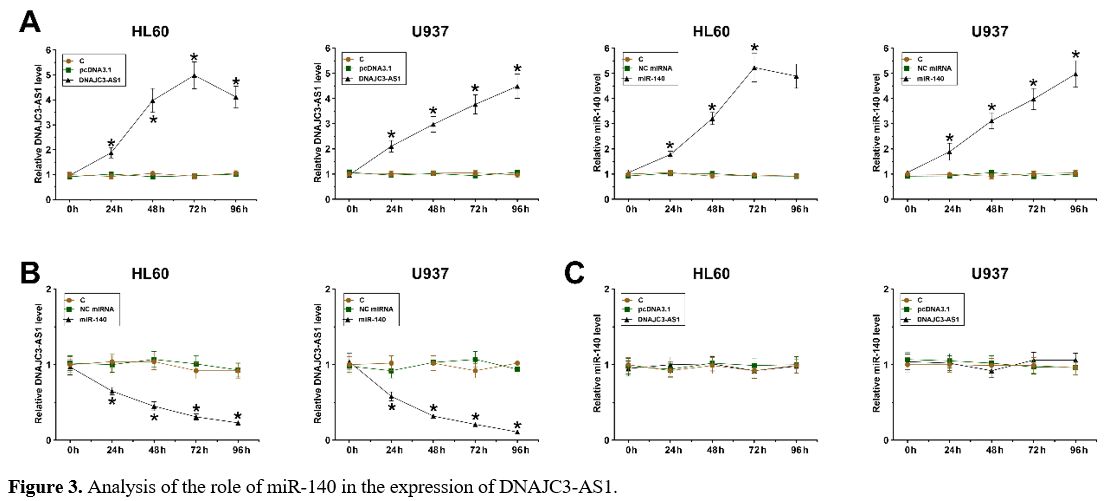 |
Figure 3. Analysis of the role of miR-140 in the expression of DNAJC3-AS1. |
The Role of DNAJC3-AS1 and miR-140 in the Proliferation of HL60 and U937 Cells.
BrdU assay was performed to explore the role of DNAJC3-AS1 and miR-140
in the proliferation of HL60 and U937 cells. DNAJC3-AS1 increased cell
proliferation, and miR-140 decreased cell proliferation. Moreover,
DNAJC3-AS1 inhibited the role of miR-140 in suppressing cell
proliferation (Figure 4, p < 0.01). Therefore, DNAJC3-AS1 is likely downstream of miR-140 in regulating AML cell proliferation.
BrdU
assay was performed to explore the role of DNAJC3-AS1 and miR-140 in
the proliferation of HL60 and U937 cells. Experiments were repeated in
three biological replicates, and the mean +/- SD values of these three
were presented. *, p < 0.01.
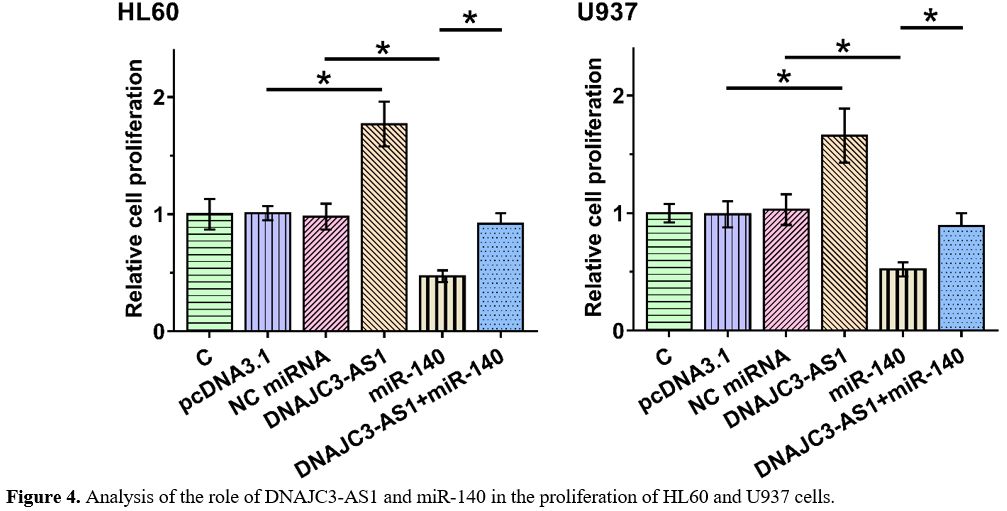 |
Figure 4. Analysis of the role of DNAJC3-AS1 and miR-140 in the proliferation of HL60 and U937 cells. |
Discussion
This
study explored the crosstalk between two ncRNAs DNAJC3-AS1 and miR-140
in AML. We showed that DNAJC3-AS1 could be targeted by miR-140 in AML
cells.
Previous studies have reported the oncogenic role of
DNAJC3-AS1 in several types of cancer, including colorectal cancer,
renal cell carcinoma, and osteosarcoma.[14-16]
DNAJC3-AS1 is upregulated in colorectal cancer to interact with the
EGFR pathway, thereby regulating fatty acid synthase to promote cancer
progression.[14] In another study, DNAJC3-AS1 was found to regulate the miR-27a-3p/PRDM14 axis to promote the development of tumors.[15] In osteosarcoma, DNAJC3-AS1 interacts with its sense-cognate gene DNAJC3 to accelerate tumor progression.[16]
Therefore, DNAJC3-AS1 could interact with different tumor suppressive
and oncogenic factors in different cancers to play an oncogenic role.
In this study, we first reported the upregulation of DNAJC3-AS1 in AML.
Overexpression of DNAJC3-AS1 led to the increased proliferation of AML
cells. Therefore, DNAJC3-AS1 also plays an oncogenic role in AML.
However, the mechanism that mediates the increased expression levels of
DNAJC3-AS1 in AML is unknown. Future studies on this topic are still
needed.
MiR-140 has been characterized as a tumor suppressor in
many types of cancer, and it targets different genes in different
cancers to inhibit cancer growth and/metastasis.[17]
For instance, decreased expression levels of miR-140 were observed in
esophageal cancer, and it regulates the wnt/β-catenin pathway by
targeting ZEB2, leading to inhibited cancer development.[17]
Furthermore, MiR-140 is also downregulated in lung cancer, and it
inhibits the growth and metastasis of tumors by downregulating
insulin-like growth factor 1 receptors through direct targeting.[18]
In addition, the expression of miR-140 is also inhibited in gastric
cancer, where it targets TES1 to suppress tumor metastasis and growth. However,
the involvement of miR-140 in AML has not been reported in previous
studies. In this study, we observed the downregulation of miR-140 in
AML and the inhibitory effects of miR-140 on AML cell proliferation.
Therefore, miR-140 is a tumor suppressor in AML.
Interestingly,
we detected DNAJC3-AS1 in both nuclear and cytoplasm of AML cells.
Moreover, miR-140 directly interacted with DNAJC3-AS1, and
overexpression of miR-140 decreased the expression levels of
DNAJC3-AS1. Because mature miRNAs are only in the cytoplasm and
DNAJC3-AS1 can be detected in the cytoplasm, we concluded that
DNAJC3-AS1 could be targeted by miR-140. Therefore, besides
protein-coding genes, miR-140 can also target oncogenic lncRNAs to
suppress cancers.
Conclusions
miR-140
is downregulated in AML, and DNAJC3-AS1 is upregulated in AML.
Therefore, MiR-140 may target DNAJC3-AS1 to suppress AML cell
proliferation.
Ethics Approval and Consent to Participate
This
study was approved by the Ethics Committee of the First Affiliated
Hospital of Shandong First Medical University & Shandong Provincial
Qianfoshan Hospital and all the patients or parents/guardians of
patients provided written informed consent.
References
- WDöhner H, Weisdorf D J, Bloomfield C D. Acute
Myeloid Leukemia [J]. The New England Journal of Medicine, 2015,
373(12): 1136-1152. https://doi.org/10.1056/NEJMra1406184 PMid:26376137
- Weber G F. Molecular mechanisms of metastasis [J]. Cancer Letters, 2008, 270(2): 181-190. https://doi.org/10.1016/j.canlet.2008.04.030 PMid:18522865
- Shallis
R M, Wang R, Davidoff A, Ma X, Zeidan A M. Epidemiology of acute
myeloid leukemia: Recent progress and enduring challenges [J]. Blood
Reviews, 2019, 36(70-87. https://doi.org/10.1016/j.blre.2019.04.005 PMid:31101526
- Padilha
S L, Souza E J, Matos M C, Domino N R. Acute myeloid leukemia: survival
analysis of patients at a university hospital of Paraná [J]. Revista
Brasileira de Hematologia e Hemoterapia, 2015, 37(1): 21-27. https://doi.org/10.1016/j.bjhh.2014.11.008 PMid:25638763 PMCid:PMC4318841
- Oran
B, Weisdorf D J. Survival for older patients with acute myeloid
leukemia: a population-based study [J]. Haematologica, 2012, 97(12):
1916-1924. https://doi.org/10.3324/haematol.2012.066100 PMid:22773600 PMCid:PMC3590098
- Short
N J, Konopleva M, Kadia T M, Borthakur G, Ravandi F, DiNardo C D, Daver
N. Advances in the Treatment of Acute Myeloid Leukemia: New Drugs and
New Challenges [J]. Cancer Discovery, 2020, 10(4): 506-525. https://doi.org/10.1158/2159-8290.CD-19-1011 PMid:32014868
- Finn
L, Dalovisio A, Foran J. Older Patients With Acute Myeloid Leukemia:
Treatment Challenges and Future Directions [J]. The Ochsner Journal,
2017, 17(4): 398-404.
- Lee Y T, Tan Y J,
Oon C E. Molecular targeted therapy: Treating cancer with specificity
[J]. European Journal of Pharmacology, 2018, 834(188-196. https://doi.org/10.1016/j.ejphar.2018.07.034 PMid:30031797
- Kayser
S, Levis M J. Advances in targeted therapy for acute myeloid leukaemia
[J]. British Journal of Haematology, 2018, 180(4): 484-500. https://doi.org/10.1111/bjh.15032 PMid:29193012 PMCid:PMC5801209
- Terao T, Minami Y. Targeting Hedgehog (Hh) Pathway for the Acute Myeloid Leukemia Treatment [J]. Cells, 2019, 8(4): https://doi.org/10.3390/cells8040312 PMid:30987263 PMCid:PMC6523210
- Liang
H, Zheng Q L, Fang P, Zhang J, Zhang T, Liu W, Guo M, Robinson C L,
Chen S B, Chen X P, Chen F P, Zeng H. Targeting the PI3K/AKT pathway
via GLI1 inhibition enhanced the drug sensitivity of acute myeloid
leukemia cells [J]. Scientific Reports, 2017, 7(40361. https://doi.org/10.1038/srep40361 PMid:28098170 PMCid:PMC5241777
- Arun
G, Diermeier S D, Spector D L. Therapeutic Targeting of Long Non-Coding
RNAs in Cancer [J]. Trends in Molecular Medicine, 2018, 24(3): 257-277.
https://doi.org/10.1016/j.molmed.2018.01.001 PMid:29449148 PMCid:PMC5840027
- Chakraborty
C, Sharma A R, Sharma G, Doss C G P, Lee S S. Therapeutic miRNA and
siRNA: Moving from Bench to Clinic as Next Generation Medicine [J].
Molecular Therapy Nucleic Acids, 2017, 8(132-143. https://doi.org/10.1016/j.omtn.2017.06.005 PMid:28918016 PMCid:PMC5496203
- Tang
Y, Tang R, Tang M, Huang P, Liao Z, Zhou J, Zhou L, Su M, Chen P, Jiang
J, Hu Y, Zhou Y, Liao Q, Zeng Z, Xiong W, Chen J, Nie S. LncRNA
DNAJC3-AS1 Regulates Fatty Acid Synthase via the EGFR Pathway to
Promote the Progression of Colorectal Cancer [J]. Frontiers in
Oncology, 2020, 10(604534. https://doi.org/10.3389/fonc.2020.604534 PMid:33604287 PMCid:PMC7885865
- Na
X Y, Hu X Q, Zhao Y, Hu C H, Shang X S. LncRNA DNAJC3-AS1 functions as
oncogene in renal cell carcinoma via regulation of the
miR-27a-3p/PRDM14 axis [J]. European Review for Medical and
Pharmacological Sciences, 2021, 25(3): 1291-1301.
- Liang
R, Liu Z, Chen Z, Yang Y, Li Y, Cui Z, Chen A, Long Z, Chen J, Lu J,
Huang B, Li Q. Long noncoding RNA DNAJC3-AS1 promotes osteosarcoma
progression via its sense-cognate gene DNAJC3 [J]. Cancer Medicine,
2019, 8(2): 761-772. https://doi.org/10.1002/cam4.1955 PMid:30652414 PMCid:PMC6382712
- Yang
S, Li X, Shen W, Hu H, Li C, Han G. MicroRNA-140 Represses Esophageal
Cancer Progression via Targeting ZEB2 to Regulate Wnt/β-Catenin Pathway
[J]. The Journal of Surgical Research, 2021, 257(267-277. https://doi.org/10.1016/j.jss.2020.07.074 PMid:32862055
- Yuan
Y, Shen Y, Xue L, Fan H. miR-140 suppresses tumor growth and metastasis
of non-small cell lung cancer by targeting insulin-like growth factor 1
receptor [J]. PloS one, 2013, 8(9): e73604. https://doi.org/10.1371/journal.pone.0073604 PMid:24039995 PMCid:PMC3769283
[TOP]