Flavio De Maio1,2, Delia Mercedes Bianco2 and Giovanni Delogu2,3.
1 Dipartimento
di Scienze di Laboratorio e Infettivologiche, Fondazione Policlinico
Universitario “A. Gemelli”, IRCCS, Rome, Italy.
2
Dipartimento di Scienze biotecnologiche di base, cliniche
intensivologiche e perioperatorie – Sezione di Microbiologia,
Università Cattolica del Sacro Cuore, Rome, Italy.
3 Mater Olbia Hospital, Olbia, Italy.
Correspondence to: Flavio
De Maio. Dipartimento di Scienze di Laboratorio e Infettivologiche,
Fondazione Policlinico Universitario “A. Gemelli”, IRCCS, Rome, Italy.
E-mail:
flavio.demaio@unicatt.it
ublished: March 1, 2022
Received: September 20, 2021
Accepted: February 11, 2022
Mediterr J Hematol Infect Dis 2022, 14(1): e2022021 DOI
10.4084/MJHID.2022.021
This is an Open Access article distributed
under the terms of the Creative Commons Attribution License
(https://creativecommons.org/licenses/by-nc/4.0),
which permits unrestricted use, distribution, and reproduction in any
medium, provided the original work is properly cited.
|
Abstract
Since
the emergence of Severe Acute Respiratory Syndrome Coronavirus 2
(SARS-CoV-2) at the end of 2019, a number of medications have been used
to treat the infection and the related Coronavirus disease – 19
(COVID-19). Some of the administered drugs were tested or used
in practice only on the basis of biological plausibility; a promising
strategy was to target the host immune response, with host directed
therapies (HDTs), to reduce systemic hyperinflammation and
hypercytokinemia responsible for additional tissue damage. We summarize the treatments against SARS-CoV-2 and underline their possible effects on Mycobacterium tuberculosis (Mtb) infection. Both SARS-CoV-2 and Mtb
respiratory infections impair the host’s immune response. Furthermore,
little research has been conducted on the impact of medicaments used to
counteract COVID-19 disease in patients with Latent Tuberculosis
Infection (LTBI). A number of these drugs may modulate host immune
response by modifying LTBI dynamic equilibrium, favoring either the
host or the bacteria.
|
Introduction
COVID-19
pandemic has shown a significant disruptive impact on Tuberculosis (TB)
services, with negative effects on prompt diagnosis, treatment and
immunization.[1,2] Pressure on laboratories and
pharmaceutical industries led to the readaptation of many TB labs to
detect SARS-CoV-2 as well as Bacillus Calmette-Guérin (BCG) shortages
and consequent decrease of newborn vaccinations.[3] Estimates indicate a 25% drop in the global BCG coverage and an increase in pediatric deaths ranging from 0.5% to 17%.[4]
In
several countries, reports suggest a decline in case notification in
the last few months due to massive cancellation of routine health
services in many
settings.[5-7] Although it has
been noted that many of the preventive measures implemented to reduce
SARS-CoV-2 incidence also have a clear benefit on reducing Mycobacterium tuberculosis (Mtb)
transmission, 2020 saw the first year-over-year increase in TB deaths
from 2005, regardless of physical distancing and PPE (personal
protective equipment) wearing measures.[2,8]
In
Canada, the pandemic significantly affected latent TB infection (LTBI)
and active TB treatment, leading to ineffective measures for TB
elimination.[9] In Spain, newly diagnosed TB
patients had more extended pulmonary disease, moreover there was a rise
in household transmission probably due to anti-COVID-19 measures.[10]
Also, in England, it has been observed a fall in rates of TB treatment
initiation during the period of government-imposed lockdown (March
23–May 10, 2020), and an increase of cases of disseminated TB during
the COVID-19 pandemic.[11] All this makes it
important to evaluate the measures against COVID-19 globally and not
only considering the pathologies related to SARS-CoV-2,
The
COVID-19 emergence prompted the scientific community to focus on
determining the mechanisms of transmission, the identification of
virulence factors of SARS-CoV-2 and the development of suitable
therapies.[12] Therapeutical management of COVID-19
is in constant change, and treatment guidelines are readily updated
based on scientific evidence and experts’ opinion (National Institutes
of Health, n.d.) as we entered in an era of “hype-based medicine”,[13]
the long forgotten eminence-based medicine regained importance as the
number of trials on possible therapies multiplied, some of them causing
overnight changes in the management of COVID-19 patients.[14]
The lack of antiviral therapies and the rapid spread of the
infection convinced investigators and pharmaceutical companies to focus
on the development of vaccines,[15] able to
induce neutralizing antibodies against SARS-CoV-2 Spike protein in
naive subjects. The developed vaccines do not only trigger a humoral
response against the protein, but they impact all the components of the
immune response.
As most of the therapies used against COVID-19
disease therapies do not target SARS-CoV-2, but aim to regulate the
host immune response[16,17] it is reasonable to consider the long-term effects of these therapies on subjects with latent TB infection (LTBI).
In this commentary, we aim to summarize treatments against SARS-CoV-2 and underline their possible effects on Mtb
infection highlighting likely “side” effects that could help to contain
virus-mediated damage and, conversely, prompt mycobacterial replication
in both early infection or during Mtb latency.
Therapies Against SARS-CoV-2 Infection
SARS-CoV-2
represents the biggest therapeutic challenge of our century. At
present, approximately 2900 clinical trials have been registered,[14] designing new molecules and repurposing existing drugs based on the virus biology and pathogenesis.
Therapeutical
approaches range from convalescent plasma of people who have recovered
from COVID-19, to medications which are commonly used to treat
autoimmune or inflammatory diseases as well as drugs used to treat
other infections.[18,19]
Pharmaceuticals used
for COVID‑19 target different pathogenetic mechanisms, with the aim of
a) blocking viral replication, summarized in points 1-3 of the Figure 1, and b) reducing tissue damage, modulating the immune responses, and preventing over-inflammation (Figure 1, points 4-6).
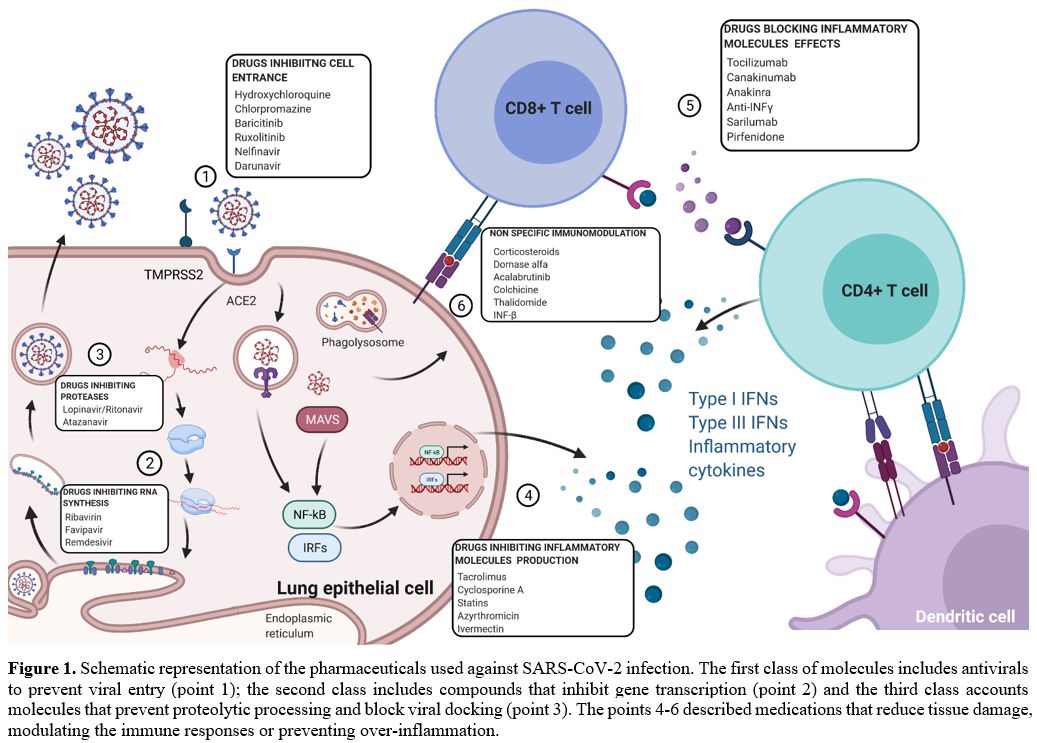 |
Figure 1. Schematic
representation of the pharmaceuticals used against SARS-CoV-2
infection. The first class of molecules includes antivirals to prevent
viral entry (point 1); the second class includes compounds that inhibit
gene transcription (point 2) and the third class accounts molecules
that prevent proteolytic processing and block viral docking (point 3).
The points 4-6 described medications that reduce tissue damage,
modulating the immune responses or preventing over-inflammation. |
The first class includes antivirals to prevent spike-protein-mediated cell fusion, thus blocking viral entry (Figure 1, point 1), inhibit gene transcription (Figure 1, point 2) or prevent proteolytic processing and block viral docking (Figure 1, point 3), as explained in Table 1.[20-23]
Interestingly, several agents show no effects on SARS-CoV-2 even
though they were described to have activity against other infections.[24]
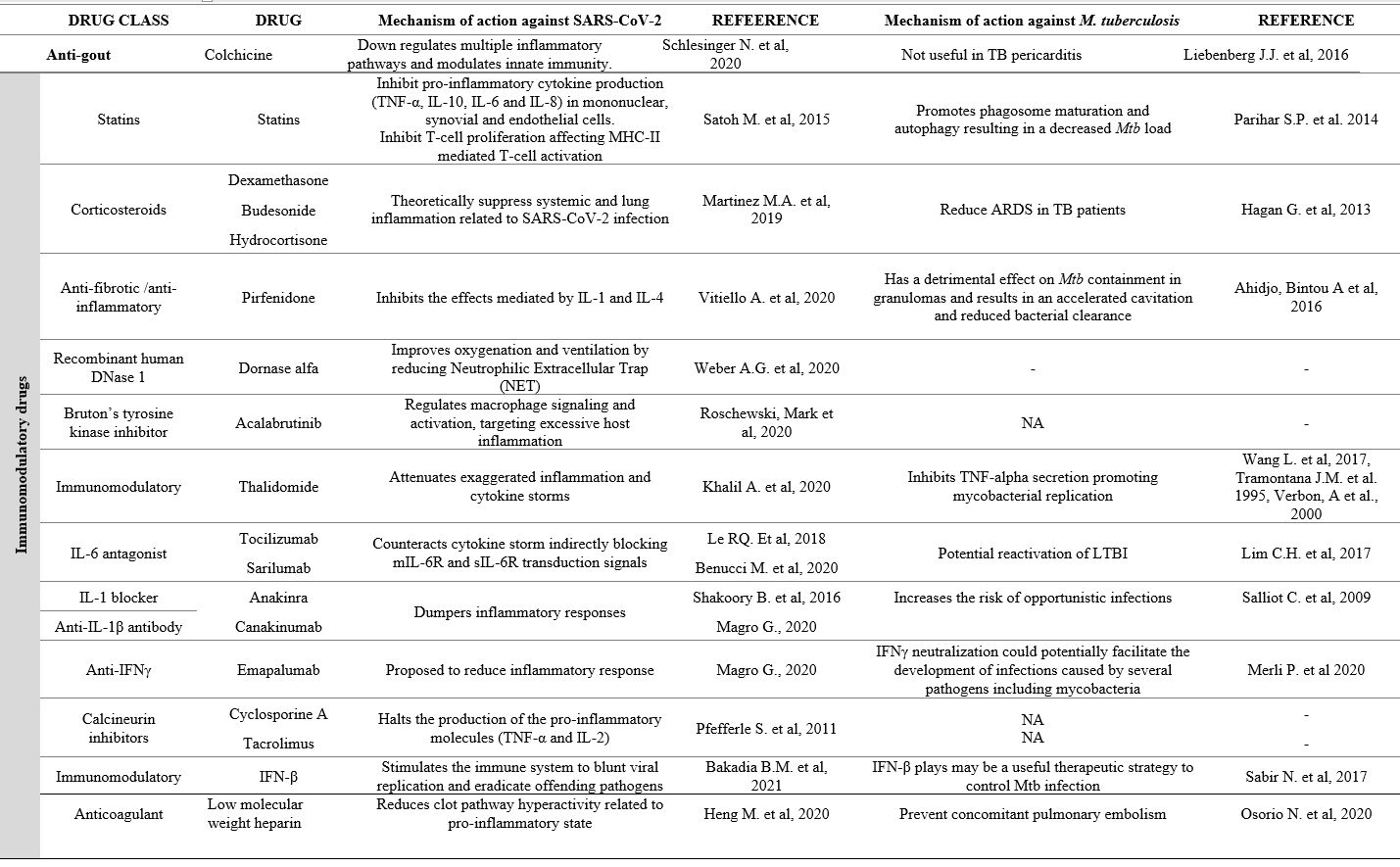 |
Table 1. Summary reporting
experimental evidence of the impact of drugs used against SARS-CoV-2
infection on Mycobacterium tuberculosis infection. |
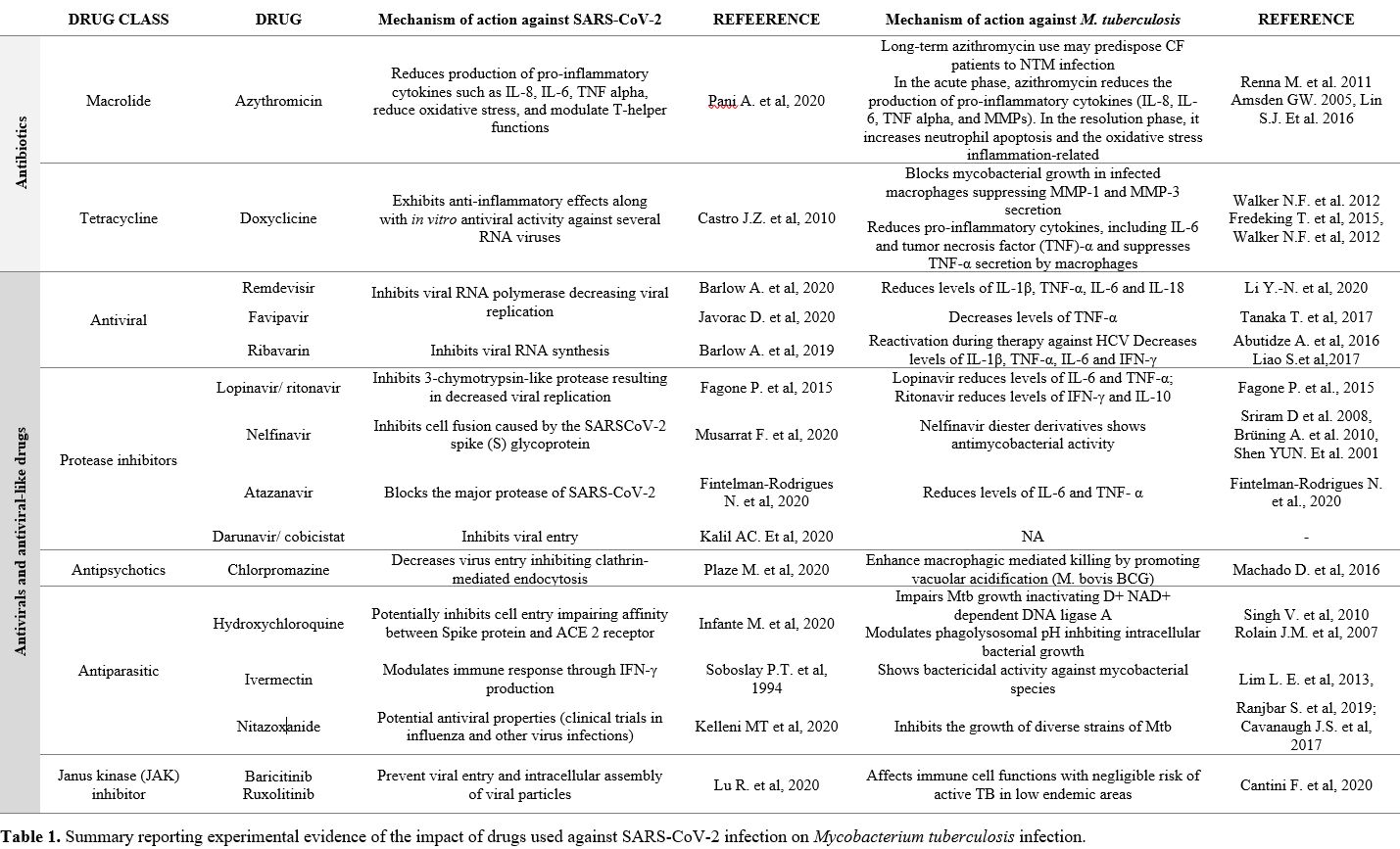 |
|
SARS-CoV-2
infection causes an overproduction of type I interferons triggering the
transcription of several genes and the recruitment of CD4+ T helper
lymphocytes, responsible for the Th1/Th2 response.[25]
For this reason, immunomodulators (corticosteroids, interferons,
monoclonal antibodies against inflammatory cytokines) have been
suggested, and largely used, to reduce the over-inflammation that is
responsible for several systemic disease manifestations.
However,
the NHS Panel failed to evaluate the real role of some of these
therapies due to insufficient evidence to recommend either for or
against their use.[26]
Examples of drugs in this category are IL-1 inhibitors, colchicine, the antiparasitic agent ivermectin,[12] and thalidomide.[27] Some others are currently recommended as IL-6 inhibitors and Janus Kinase inhibitors (refer to Table 1 and Figure 1, point 4 to 6).
Although,
the use of immunomodulatory treatments had an immediate impact on the
care of patients infected with SARS-CoV-2, their long-term effects are
unknown.
Impact of the Therapies Against SARS-CoV-2 on Mycobacterium tuberculosis
Mtb
infection represents a classical model of persistent infection, a
situation in which a microorganism can persist indefinitely within the
host,[28,29] establishing an equilibrium between the
pathogen and the host immune response whose modification could increase
the risk of relapse and disease. Indeed, host immune response can
limit Mtb spread, after macrophages killing evasion, creating a multicellular structure known as granuloma,[30] which entraps mycobacteria that persist in a heterogeneous range of states.[31] In the last decades, to deal with the emergence of Mtb strains resistant anti-TB drugs (MDR/RR-Mtb and XDR-Mtb), a novel approach has been proposed targeting the host and so named host directed therapies (HDTs).[32–35]
HDTs can support antimycobacterial host response at different stages:
a) perturbating granuloma integrity to enhance drug penetration; b)
modifying autophagy or phagosome maturation to increase intracellular
killing; c) promoting cell-mediated response; d) inducing antimicrobial
peptides and controlling inflammation response by avoiding tissue
damage.[36] While the use of HDTs seem to support anti-TB treatment in symptomatic individuals,[37] no data nor anecdotal knowledge support the use of such therapies in people with asymptomatic or subclinical infection.[38]
In other words, it is undeniable that some immunomodulatory treatments may alter the host-Mtb
equilibrium, favoring either the host or the bacteria. In this
scenario, we cannot exclude that those immunomodulatory therapies used
against COVID-19 may have a negative effect on infected individuals
causing symptomatic TB.
A recent paper highlighted the relationship between SARS-CoV-2 and Mtb
infection, showing that asymptomatic SARS-CoV-2 seropositive
individuals with a positive IGRA exhibited heightened levels of
humoral, cytokine production, and systemic inflammation compared to
individuals negative for Mtb infection.[39] Mtb
is apparently able to modulate the host immune response in
SARS-CoV-2-infected individuals. Furthermore, various clinical cases
describe TB reactivation following SARS-CoV-2 infection confirming the
concerns that COVID-19 associated CD4+ T-cell depletion or altered
T-cell function can have similar implications as HIV for TB disease
progression, promoting the development of active TB.[25,40] Moreover, some studies highlighted a higher probability to develop severe disease in patients with SARS-CoV-2 / Mtb co-infection compared to COVID-19 patients.[41,42] Unfortunately, we have little information on TB occurrence after COVID-19 treatments.[43]
On the other hand, there was a delay in the onset of the pandemics in
many countries endemic for TB. Moreover, those countries showed lower
COVID-19’s severe cases and SARS-CoV-2 related-mortality.[44] Intriguingly, one of the variables that was mathematically linked to COVID-19 low spread was BCG vaccination,[45]
which is known to stimulate non-specific heterologous immune responses
inducing cross-protective effects toward non-tuberculosis-related
diseases, included SARS-CoV-2.[15,46,47]
Indeed, numerous clinical trials are currently registered to update on
the benefits of BCG vaccinations against SARS-CoV-2 exposure.[15,47]
We can classify therapies used against COVID-19 based on their activity on Mtb infection in four main drug classes: a) drugs acting directly on Mtb (Figure 2, point 1); b) drugs that modify phagosome acidification (Figure 2, point 2); c) drugs with adjuvant function that can indirectly modulate the infection (Figure 2, point 3) and d) drugs with immunomodulatory activity (Figure 2, point 4). Although many anti-COVID-19 pharmaceutics appeared to impair mycobacterial growth in in vitro experiments, (Figure 2, point 1),[48–51] we focus our attention on their immunomodulatory effects (Table 1).
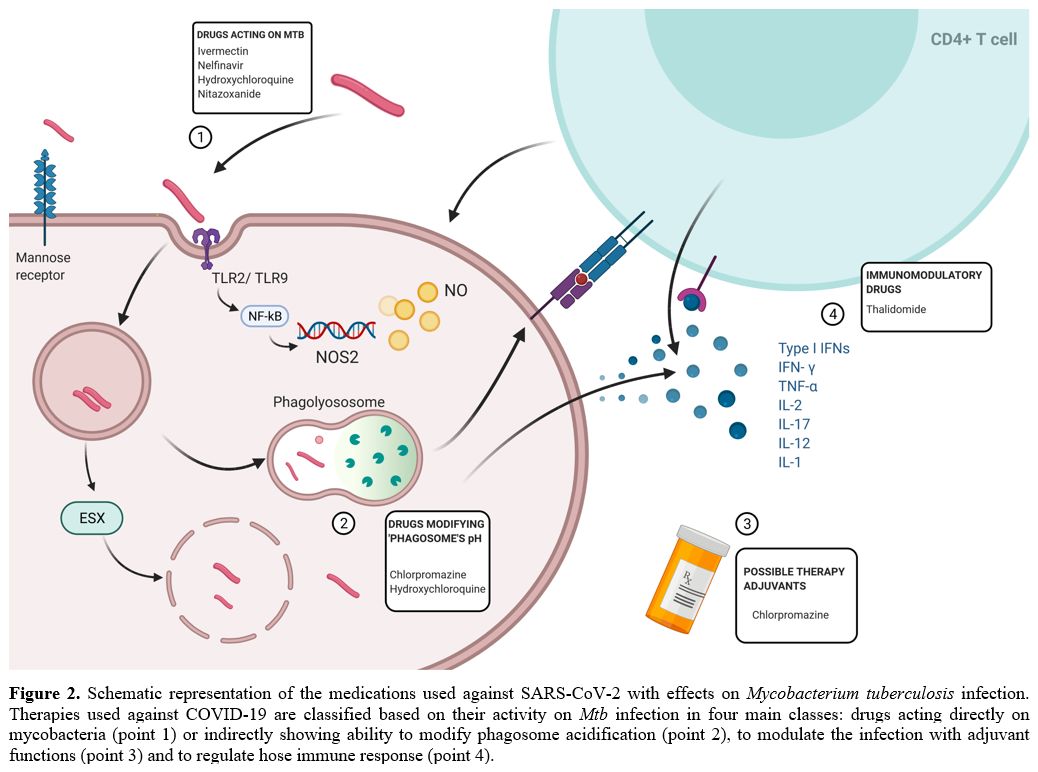 |
Figure 2. Schematic
representation of the medications used against SARS-CoV-2 with effects
on Mycobacterium tuberculosis infection. Therapies used against
COVID-19 are classified based on their activity on Mtb
infection in four main classes: drugs acting directly on mycobacteria
(point 1) or indirectly showing ability to modify phagosome
acidification (point 2), to modulate the infection with adjuvant
functions (point 3) and to regulate hose immune response (point 4). |
Hydroxychloroquine inactivates mycobacterial NAD+ dependent DNA ligase A[52]
and modulates phagolysosome, reducing intracellular mycobacterial
growth.[48] Similarly, chlorpromazine, an antipsychotic drug, has been
observed to have antimycobacterial activity and to promote macrophagic
killing by increasing phagosome acidification.[53] Acidification may though differently affect phagosomes at different stages of maturation.[54] Moreover, Mtb itself can influence phagosome maturation potentially counteracting these drugs.[55]
Nitazoxanide, which has been also suggested to modulate immune response inducing interferon-γ,[50,56] inhibits intracellular Mtb growth while amplifying Mtb-induced gene expression.
Thalidomide represents a compound that has been tested against Mtb
infection showing a detrimental effect on infection control due to
TNF-α inhibition with consequent increase in mycobacterial replication[57,58] (Figure 2 and Table 1).
Interestingly, several drugs that have been proposed against SARS-CoV-2 have not been tested in vitro against Mtb infection (Table 1).
This is true for several molecules that act on the host immune system
to prevent over inflammation (which has been observed as a critical
point for the progression of SARS-CoV-2 infection). These compounds,
that dampen pro-inflammatory cytokines, could impair the fine
equilibrium between Mtb
replication and the host immune system response, thus promoting active
disease. Among them, monoclonal antibodies such as IL-6 antagonists and
antivirals have been observed to significantly modulate host cytokine
response and potentially alter host immune response versus Mtb replication.[59] Interestingly, Mtb
regulates IL-6 secretion to inhibit type I interferon signaling and
causes disease progression which appears to be associated to sigH gene expression.[60] For this reason, IL-6 antagonist could have important implications during Mtb infection.
Another example are corticosteroids that are beneficial in hospitalized COVID-19 patients,[61,62] but, conversely, could increase the risk of LTBI reactivation or progression of sub-clinical TB.
Conclusions
Given
the specific effect of COVID-19 on T-cells and for anti-COVID-19
treatments on LTBI, clinicians should consider monitoring patients with
both previous COVID-19 infection and LTBI to rapidly identify active
disease and prevent Mtb transmission.
References
- Furtado I, Aguiar A, Duarte R. Getting back on the
road towards tuberculosis elimination: lessons learnt from the COVID-19
pandemic. J Bras Pneumol. 2021;47(2):e20210123. https://doi.org/10.36416/1806-3756/e20210123 PMid:33950099 PMCid:PMC8332847
- Pai
M, Kasaeva T, Swaminathan S. Covid-19's Devastating Effect on
Tuberculosis Care - A Path to Recovery. N Engl J Med [Internet].
2022;0(0):null. https://doi.org/10.1056/NEJMp2118145 PMid:34986295
- Jain
VK, Iyengar KP, Samy DA, Vaishya R. Tuberculosis in the era of COVID-19
in India. Diabetes Metab Syndr Clin Res Rev [Internet].
2020;14(5):1439-43. https://doi.org/10.1016/j.dsx.2020.07.034 PMid:32755848 PMCid:PMC7387287
- Shaikh
N, Pelzer PT, Thysen SM, Roy P, Harris RC, White RG. Impact of COVID-19
Disruptions on Global BCG Coverage and Paediatric TB Mortality: A
Modelling Study. Vaccines. 2021 Oct;9(11). https://doi.org/10.3390/vaccines9111228 PMid:34835161 PMCid:PMC8624525
- Malik
AA, Safdar N, Chandir S, Khan U, Khowaja S, Riaz N, et al. Tuberculosis
control and care in the era of COVID-19. Health Policy Plan.
2020;35(8):1130-2. https://doi.org/10.1093/heapol/czaa109 PMid:32832996 PMCid:PMC7499582
- Janice
K. Louie, Rocio Agraz-Lara LR, Felix Crespin, Lisa Chen 1, Graves S.
Tuberculosis-Associated Hospitalizations and Deaths after COVID-19
ShelterIn-Place, San Francisco, California, USA. Emerg Infect Dis.
2021;27:2227-9. https://doi.org/10.3201/eid2708.210670 PMid:34287142 PMCid:PMC8314834
- Marwah
V, Peter DK, Ajai Kumar T, Bhati G, Kumar A. Multidrug-resistant
tuberculosis in COVID-19: Double trouble. Med J Armed Forces India
[Internet]. 2021;77:S479-82. https://doi.org/10.1016/j.mjafi.2021.05.002 PMid:34334915 PMCid:PMC8313087
- Finn
McQuaid C, McCreesh N, Read JM, Sumner T, Houben RMGJ, White RG, et al.
The potential impact of COVID-19-related disruption on tuberculosis
burden. Eur Respir J. 2020;56(2). https://doi.org/10.1183/13993003.01718-2020 PMid:32513784 PMCid:PMC7278504
- Geric
C, Saroufim M, Landsman D, Richard J, Benedetti A, Batt J, et al.
Impact of Covid-19 on Tuberculosis Prevention and Treatment in Canada:
a multicentre analysis of 10,833 patients. J Infect Dis. 2021 Dec. https://doi.org/10.1093/infdis/jiab608 PMid:34919700 PMCid:PMC8755327
- Aznar
ML, Espinosa-Pereiro J, Saborit N, Jové N, Sánchez Martinez F,
Pérez-Recio S, et al. Impact of the COVID-19 pandemic on tuberculosis
management in Spain. Int J Infect Dis IJID Off Publ Int Soc Infect Dis.
2021 Jul;108:300-5. https://doi.org/10.1016/j.ijid.2021.04.075 PMid:33930543 PMCid:PMC8078060
- Barrett
J, Painter H, Rajgopal A, Keane D, John L, Papineni P, et al. Increase
in disseminated TB during the COVID-19 pandemic. Vol. 25, The
international journal of tuberculosis and lung disease : the official
journal of the International Union against Tuberculosis and Lung
Disease. France; 2021. p. 160-6. https://doi.org/10.5588/ijtld.20.0846 PMid:33656432
- Tu
YF, Chien CS, Yarmishyn AA, Lin YY, Luo YH, Lin YT, et al. A review of
sars-cov-2 and the ongoing clinical trials [Internet]. Vol. 21,
International Journal of Molecular Sciences. 2020. p. 2657. https://doi.org/10.3390/ijms21072657 PMid:32290293 PMCid:PMC7177898
- Pearson H. How COVID broke the evidence pipeline. Nature. 2021;593(7858):182-5. https://doi.org/10.1038/d41586-021-01246-x PMid:33981057
- No authors listed. Evidence-based medicine: how COVID can drive positive change. Vol. 593, Nature. England; 2021. p. 168. https://doi.org/10.1038/d41586-021-01255-w PMid:33981058
- Gong
W, Aspatwar A, Wang S, Parkkila S, Wu X. COVID-19 pandemic: SARS-CoV-2
specific vaccines and challenges, protection via BCG trained immunity,
and clinical trials. Expert Rev Vaccines. 2021 Jul;20(7):857-80. https://doi.org/10.1080/14760584.2021.1938550 PMid:34078215 PMCid:PMC8220438
- Hu B, Guo H, Zhou P, Shi ZL. Characteristics of SARS-CoV-2 and COVID-19. Nat Rev Microbiol [Internet]. 2021;19(3):141-54. https://doi.org/10.1038/s41579-020-00459-7 PMid:33024307 PMCid:PMC7537588
- Petersen
E, Koopmans M, Go U, Hamer DH, Petrosillo N, Castelli F, et al.
Personal View Comparing SARS-CoV-2 with SARS-CoV and influenza
pandemics. Lancet Infect Dis [Internet]. 2020;20(9):e238-44. https://doi.org/10.1016/S1473-3099(20)30484-9
- Nitulescu
GM, Paunescu H, Moschos SA, Petrakis D, Nitulescu G, Ion GND, et al.
Comprehensive analysis of drugs to treat SARS-CoV-2 infection:
Mechanistic insights into current COVID-19 therapies (Review). Int J
Mol Med. 2020;46(2):467-88. https://doi.org/10.3892/ijmm.2020.4608 PMid:32468014 PMCid:PMC7307820
- Joyner
MJ, Wright RS, Fairweather DL, Senefeld JW, Bruno KA, Klassen SA, et
al. Early safety indicators of COVID-19 convalescent plasma in 5,000
patients. medRxiv. 2020;130(9):4791-7. https://doi.org/10.1101/2020.05.12.20099879 PMid:32511566 PMCid:PMC7274247
- Sarma
P, Shekhar N, Prajapat M, Avti P, Kaur H, Kumar S, et al. In-silico
homology assisted identification of inhibitor of RNA binding against
2019-nCoV N-protein (N terminal domain). J Biomol Struct Dyn. 2021
May;39(8):2724-2732. http://doi.org/10.1080/07391102.2020.1753580 PMID: 32266867
- O'keefe
BR, Giomarelli B, Barnard DL, Shenoy SR, Chan PKS, Mcmahon JB, et al.
Broad-Spectrum In Vitro Activity and In Vivo Efficacy of the Antiviral
Protein Griffithsin against Emerging Viruses of the Family
Coronaviridae. J Virol [Internet]. 2010;84(5):2511-21. https://doi.org/10.1128/JVI.02322-09 PMid:20032190 PMCid:PMC2820936
- Plaze
M, Attali D, Petit AC, Blatzer M, Simon-Loriere E, Vinckier F, et al.
Repurposing chlorpromazine to treat COVID-19: The reCoVery study.
Encephale. 2020 Jun 1;46(3):169-72. https://doi.org/10.1016/j.encep.2020.05.006 PMid:32425222 PMCid:PMC7229964
- Infante
M, Ricordi C, Alejandro R, Caprio M, Fabbri A. Hydroxychloroquine in
the COVID-19 pandemic era: in pursuit of a rational use for prophylaxis
of SARS-CoV-2 infection. Expert Rev Anti Infect Ther. 2020; https://doi.org/10.1080/14787210.2020.1799785 PMid:32693652 PMCid:PMC7441799
- Mina
T. Kellen. Letter to the Editor Nitazoxanide/azithromycin combination
for COVID-19: A suggested new protocol for early management. Pharmacol
Res J [Internet]. 2020; https://doi.org/10.20944/preprints202004.0432.v1
- Riou
C, du Bruyn E, Stek C, Daroowala R, Goliath RT, Abrahams F, et al.
Relationship of SARS-CoV-2-specific CD4 response to COVID-19 severity
and impact of HIV-1 and tuberculosis coinfection. J Clin Invest
[Internet]. 2021;131(12). https://doi.org/10.1172/JCI149125 PMid:33945513 PMCid:PMC8203446
- National
Institutes of Health. COVID-19 Treatment Guidelines Panel. Coronavirus
Disease 2019 (COVID-19) Treatment Guidelines [Internet]. [cited 2021
Aug 12].
- Chen C, Qi F, Shi K, Li Y, Li
J, Chen Y, et al. Thalidomide Combined with Low-dose Glucocorticoid in
the Treatment of COVID-19 Pneumonia [Internet]. Preprints; 2020 [cited
2021 Aug 13].
- Kane M, Golovkina T. Common Threads in Persistent Viral Infections. J Virol. 2010;84(9):4116-23. https://doi.org/10.1128/JVI.01905-09 PMid:19955304 PMCid:PMC2863747
- Fisher RA, Gollan B, Helaine S. Persistent bacterial infections and persister cells. Nat Rev Microbiol. 2017 Aug;15(8):453-64. https://doi.org/10.1038/nrmicro.2017.42 PMid:28529326
- Russell
DG, Cardona PJ, Kim MJ, Allain S, Altare F. Foamy macrophages and the
progression of the human tuberculosis granuloma. Nat Immunol.
2009;10(9):943-8. https://doi.org/10.1038/ni.1781 PMid:19692995 PMCid:PMC2759071
- Delogu G, Sali M, Fadda G. The biology of mycobacterium tuberculosis infection. Mediterr J Hematol Infect Dis. 2013;5(1). https://doi.org/10.4084/mjhid.2013.070 PMid:24363885 PMCid:PMC3867229
- Kaufmann
SHE, Dorhoi A, Hotchkiss RS, Bartenschlager R. Host-directed therapies
for bacterial and viral infections. Nat Rev Drug Discov [Internet].
2018;17(1):35-56. https://doi.org/10.1038/nrd.2017.162 PMid:28935918 PMCid:PMC7097079
- Palucci
I, Maulucci G, De Maio F, Sali M, Romagnoli A, Petrone L, et al.
Inhibition of Transglutaminase 2 as a Potential Host-Directed Therapy
Against Mycobacterium tuberculosis. Front Immunol.
2020;10(January):1-13. https://doi.org/10.3389/fimmu.2019.03042 PMid:32038614 PMCid:PMC6992558
- Kumar
R, Kolloli A, Singh P, Vinnard C, Kaplan G, Subbian S. Thalidomide and
Phosphodiesterase 4 Inhibitors as Host Directed Therapeutics for
Tuberculous Meningitis: Insights From the Rabbit Model. Front Cell
Infect Microbiol. 2019;9:450. https://doi.org/10.3389/fcimb.2019.00450 PMid:32010638 PMCid:PMC6972508
- Costa
DL, Maiga M, Subbian S. Editorial: Host-Directed Therapies for
Tuberculosis. Front Cell Infect Microbiol [Internet]. 2021;11:736.
https://doi.org/10.3389/fcimb.2021.742053 PMid:34422685 PMCid:PMC8377667
- Kolloli
A, Kumar R, Singh P, Narang A, Kaplan G, Sigal A, et al. Aggregation
state of Mycobacterium tuberculosis impacts host immunity and augments
pulmonary disease pathology. Commun Biol. 2021 Nov;4(1):1256. https://doi.org/10.1038/s42003-021-02769-9 PMid:34732811 PMCid:PMC8566596
- Wallis
RS, Ginindza S, Beattie T, Arjun N, Likoti M, Edward VA, et al.
Adjunctive host-directed therapies for pulmonary tuberculosis: a
prospective, open-label, phase 2, randomised controlled trial. Lancet
Respir Med [Internet]. 2021;9(8):897-908. https://doi.org/10.1016/S2213-2600(20)30448-3
- Richards
AS, Sossen B, Emery JC, Horton KC, Heinsohn T, Frascella B, et al. The
natural history of TB disease-a synthesis of data to quantify
progression and regression across the spectrum. medRxiv [Internet].
2021; https://doi.org/10.1101/2021.09.13.21263499
- Rajamanickam
A, Kumar NP, Padmapriyadarsini C, Nancy A, Selvaraj N, Karunanithi K,
et al. Latent tuberculosis co-infection is associated with heightened
levels of humoral, cytokine and acute phase responses in seropositive
SARS-CoV-2 infection. J Infect [Internet]. 2021;83(3):339-46. https://doi.org/10.1016/j.jinf.2021.07.029 PMid:34329676 PMCid:PMC8316716
- Khayat
M, Fan H, Vali Y. COVID-19 promoting the development of active
tuberculosis in a patient with latent tuberculosis infection: A case
report. Respir Med Case Reports. 2021 Jan 1;32:101344. https://doi.org/10.1016/j.rmcr.2021.101344 PMid:33495728 PMCid:PMC7816563
- Mousquer
GT, Peres A, Fiegenbaum M. Pathology of TB/COVID-19 Co-Infection: The
phantom menace. Tuberculosis (Edinb). 2021 Jan;126:102020. https://doi.org/10.1016/j.tube.2020.102020 PMid:33246269 PMCid:PMC7669479
- Gao
Y, Liu M, Chen Y, Shi S, Geng J, Tian J. Association between
tuberculosis and COVID-19 severity and mortality: A rapid systematic
review and meta-analysis. Vol. 93, Journal of medical virology. 2021.
p. 194-6. https://doi.org/10.1002/jmv.26311 PMid:32687228 PMCid:PMC7405273
- Tadolini
M, Codecasa LR, García-García JM, Blanc FX, Borisov S, Alffenaar JW, et
al. Active tuberculosis, sequelae and COVID-19 co-infection: First
cohort of 49 cases. Eur Respir J. 2020;56(1). https://doi.org/10.1183/13993003.02328-2020 PMid:32586888 PMCid:PMC7315815
- Lalaoui
R, Bakour S, Raoult D, Verger P, Sokhna C, Devaux C, et al. What could
explain the late emergence of COVID-19 in Africa? New microbes new
Infect. 2020 Nov;38:100760. https://doi.org/10.1016/j.nmni.2020.100760 PMid:32983542 PMCid:PMC7508045
- Zawbaa
HM, El-Gendy A, Saeed H, Osama H, Ali AMA, Gomaa D, et al. A study of
the possible factors affecting COVID-19 spread, severity and mortality
and the effect of social distancing on these factors: Machine learning
forecasting model. Int J Clin Pract. 2021 Jun;75(6):e14116. https://doi.org/10.1111/ijcp.14116 PMCid:PMC7995223
- Covián
C, Retamal-Díaz A, Bueno SM, Kalergis AM. Could BCG Vaccination Induce
Protective Trained Immunity for SARS-CoV-2? Front Immunol [Internet].
2020;11. https://doi.org/10.3389/fimmu.2020.00970 PMid:32574258 PMCid:PMC7227382
- Giamarellos-Bourboulis
EJ, Tsilika M, Moorlag S, Antonakos N, Kotsaki A, Domínguez-Andrés J,
et al. Activate: Randomized Clinical Trial of BCG Vaccination against
Infection in the Elderly. Cell. 2020 Oct;183(2):315-323.e9. https://doi.org/10.1016/j.cell.2020.08.051 PMid:32941801 PMCid:PMC7462457
- Rolain
JM, Colson P, Raoult D. Recycling of chloroquine and its hydroxyl
analogue to face bacterial, fungal and viral infections in the 21st
century. Int J Antimicrob Agents. 2007;30(4):297-308. https://doi.org/10.1016/j.ijantimicag.2007.05.015 PMid:17629679 PMCid:PMC7126847
- Lim
LE, Vilchèze C, Ng C, Jacobs WR, Ramón-García S, Thompson CJ.
Anthelmintic avermectins kill mycobacterium tuberculosis, including
multidrug-resistant clinical strains. Antimicrob Agents Chemother.
2013;57(2):1040-6. https://doi.org/10.1128/AAC.01696-12 PMid:23165468 PMCid:PMC3553693
- Ranjbar
S, Haridas V, Nambu A, Jasenosky LD, Sadhukhan S, Ebert TS, et al.
Cytoplasmic RNA Sensor Pathways and Nitazoxanide Broadly Inhibit
Intracellular Mycobacterium tuberculosis Growth. iScience [Internet].
2019;22:299-313. https://doi.org/10.1016/j.isci.2019.11.001 PMid:31805434 PMCid:PMC6909047
- Sriram
D, Yogeeswari P, Dinakaran M, Sowmya M. Synthesis, anti-HIV and
antitubercular activities of nelfinavir diester derivatives. Biomed
Pharmacother [Internet]. 2008;62(1):1-5. https://doi.org/10.1016/j.biopha.2007.08.002 PMid:17890044
- Singh
V, Somvanshi P. Toward the Virtual Screening of Potential Drugs in the
Homology Modeled NAD+ Dependent DNA Ligase from Mycobacterium
tuberculosis. Protein Pept Lett. 2010;17(2):269-76. https://doi.org/10.2174/092986610790225950 PMid:20214650
- Machado
D, Pires D, Couto I. Ion Channel Blockers as Antimicrobial Agents ,
Efflux Inhibitors , and Enhancers of Macrophage Killing Activity
against Drug Resistant Mycobacterium tuberculosis. 2016;1-28. https://doi.org/10.1371/journal.pone.0149326 PMid:26919135 PMCid:PMC4769142
- Romagnoli
A, Petruccioli E, Palucci I, Camassa S, Carata E, Petrone L, et al.
Clinical isolates of the modern Mycobacterium tuberculosis lineage 4
evade host defense in human macrophages through eluding IL-1β-induced
autophagy article. Cell Death Dis [Internet]. 2018;9(6). https://doi.org/10.1038/s41419-018-0640-8 PMid:29795378 PMCid:PMC5967325
- Ramachandra
L, Smialek JL, Shank SS, Convery M, Boom WH, Harding C V. Phagosomal
processing of Mycobacterium tuberculosis antigen 85B is modulated
independently of mycobacterial viability and phagosome maturation.
Infect Immun. 2005 Feb;73(2):1097-105. https://doi.org/10.1128/IAI.73.2.1097-1105.2005 PMid:15664953 PMCid:PMC547092
- Iacobino
A, Giannoni F, Pardini M, Piccaro G. The Combination
Rifampin-Nitazoxanide, but Not
Rifampin-Isoniazid-Pyrazinamide-Ethambutol, Kills Dormant Mycobacterium
tuberculosis in Hypoxia at Neutral pH. Antimicrob Agents Chemother.
2019;(April):1-4. https://doi.org/10.1128/AAC.00273-19 PMid:31010861 PMCid:PMC6591638
- Verbon
A, Juffermans NP, Speelman P, Van Deventer SJH, Ten Berge IJM,
Guchelaar HJ, et al. A single oral dose of thalidomide enhances the
capacity of lymphocytes to secrete gamma interferon in healthy humans.
Antimicrob Agents Chemother. 2000;44(9):2286-90. https://doi.org/10.1128/AAC.44.9.2286-2290.2000 PMid:10952569 PMCid:PMC90059
- Wang
L, Hong Y, Wu J, Leung YK, Huang Y. Efficacy of thalidomide therapy in
pediatric Crohn's disease with evidence of tuberculosis. World J
Gastroenterol. 2017;23(43):7727-34. https://doi.org/10.3748/wjg.v23.i43.7727 PMid:29209113 PMCid:PMC5703932
- Urdahl
KB, Shafiani S, Ernst JD. Initiation and regulation of T-cell responses
in tuberculosis. Vol. 4, Mucosal Immunology. 2011. p. 288-93. https://doi.org/10.1038/mi.2011.10 PMid:21451503 PMCid:PMC3206635
- Martinez
AN, Mehra S, Kaushal D. Role of interleukin 6 in innate immunity to
Mycobacterium tuberculosis infection. J Infect Dis. 2013
Apr;207(8):1253-61. https://doi.org/10.1093/infdis/jit037 PMid:23359591 PMCid:PMC3693587
- The
RECOVERY Collaborative Group. Dexamethasone in Hospitalized Patients
with Covid-19. N Engl J Med [Internet]. 2020 Jul 17;384(8):693-704. https://doi.org/10.1056/NEJMoa2021436 PMid:32678530 PMCid:PMC7383595
- Liu
J, Zhang S, Dong X, Li Z, Xu Q, Feng H, et al. Corticosteroid treatment
in severe COVID-19 patients with acute respiratory distress syndrome. J
Clin Invest. 2020 Dec;130(12):6417-28. https://doi.org/10.1172/JCI140617 PMid:33141117 PMCid:PMC7685724
[TOP]