Gang Huang1,
Jianjun Liao1, Mingli Wang1,
Yali Huang1, Mingjie Tang1 and
Yanyan Hao2
1
Department of Hematology, Yuebei People’s Hospital, Shantou University Medical College, Shaoguan, Guangdong, 512000, China.
2 Department of Clinical Laboratory, Wenzhou Hospital of traditional Chinese Medicine, Wenzhou, Zhejiang, 325000, China.
Correspondence to:
Yanyan Hao. Department of Clinical Laboratory, Wenzhou Hospital of
Traditional Chinese Medicine, No.9, Jiaowei Road, Wenzhou, Zhejiang,
China. Tel: +86-0577-88078323. Email:
haoyanyan11217@163.com.
Published: July 1, 2022
Received: January 10, 2022
Accepted: June 5, 2022
Mediterr J Hematol Infect Dis 2022, 14(1): e2022048 DOI
10.4084/MJHID.2022.048
This is an Open Access article distributed
under the terms of the Creative Commons Attribution License
(https://creativecommons.org/licenses/by-nc/4.0),
which permits unrestricted use, distribution, and reproduction in any
medium, provided the original work is properly cited.
|
Abstract
Mantle
cell lymphoma (MCL) is an aggressive lymphoid malignancy with a poor
prognosis. Ubiquitin-specific peptidase 9, X-linked (USP9X), has been
associated with multiple physiological pathways and regulates various
cellular activities. In this study, we explored the role of USP9X in
MCL in vitro and in vivo.
USP9X was verified to be increased in peripheral blood mononuclear
cells (PBMCs) of MCL patients and MCL cells. Moreover, CCND1 and SOX11
were also upregulated in PBMCs of MCL patients. The positive
correlation between USP9X and CCND1, USP9X and SOX11, and CCND1 and
SOX11 were identified. Further, USP9X overexpression and knockdown
were performed in MCL cells. We proved that USP9X overexpression
promoted proliferation and cell cycle and suppressed cell apoptosis in
MCL cells. Upregulation of angiogenesis and cell migration were induced
by USP9X overexpression in MCL cells. However, the USP9X knockdown
showed opposite effects. In addition, USP9X was discovered to decrease
Cyclin D1 (CCND1)-mediated SOX11 expression in MCL cells. We
demonstrated that SOX11 overexpression reversed USP9X
knockdown-mediated angiogenesis in MCL cells. Besides, tumor formation
was inhibited by USP9X knockdown in mice in vivo. In conclusion, these
results revealed that USP9X promoted tumor angiogenesis in MCL via
increasing CCND1- mediated SOX11.
|
Introduction
Mantle
cell lymphoma (MCL) is a relatively rare subtype of highly aggressive
B-cell lymphoma with approximately 7% of the non-Hodgkin lymphoma
incidence rate.[1] Pathologically, it is featured by the chromosomal translocation t (11;14) (q13; q32).[2] MCL has a poor prognosis compared with other types of non-Hodgkin lymphoma, with a median survival time of only 3-5 years.[3]
When most patients are diagnosed with stage III-IV, tumor infiltration
usually occurs in lymph nodes, bone marrow, spleen, peripheral blood,
and digestive tract.[4,5] In MCL, tumor cells can
shape their microenvironment and activate a complex network of
chemokines to promote tumor progression. For example, tumor stroma can
increase endothelial cell proliferation and microvascular formation and
enhance the aggressiveness of MCL.[6] Although a series of novel treatment approaches are applied to the improvement of survival rates of MCL patients,[7] about half of patients still suffer from recurrent MCL within 10 years.[8] Thus, treatment targets of MCL are necessary to be further explored.
Ubiquitin-specific
peptidase 9, X-linked (USP9X), acts as a member of the USP family to be
involved in multiple physiological pathways and regulate a variety of
cellular activities.[9-11] For example, USP9X was
highly expressed in pancreatic cancer, promoting epithelial mesenchymal
transformation and inhibiting cell apoptosis.[12,13] Moreover, USP9X was associated with regulating tumor microenvironment and angiogenesis in breast cancer.[14]
Additionally, it has been reported that USP9X expression was high in
human invasive B-cell lymphoma samples and enhanced the chemoresistance
of invasive B-cell lymphoma.[15] However, the underlying mechanism function of USP9X in MCL is still unclear.
CCND1, as a cell cycle regulatory protein, is located in chromosome 11q13 and directly regulated by p53.[16-18] Its upregulation was closely related to more advanced tumors and tumor metastasis.[19,20] Furthermore, CCND1 participated in regulating the malignant progression of MCL.[21] USP9X was proved to upregulate the expression of cell cycle-related protein CCND1,[22] suggesting that USP9X might function in MCL through CCND1.
Here,
this study aims to investigate the effects of USP9X on the development
of MCL. In addition, we confirmed the specific mechanism of USP9X on
MCL through CCND1 in MCL, which provides a potential therapeutic target
for MCL.
Materials and Methods
Clinical sample.
All specimens were obtained from 50 treatment-naive MCL patients and 20
healthy volunteers. Informed consent was gained from all volunteers.
This study was approved by the World Medical Association Declaration of
Helsinki and Yuebei people’s Hospital. Ficoll gradient centrifugation
acquired peripheral blood mononuclear cells (PBMCs) of all volunteers,
then PBMCs were purified through anti-CD19 magnetic beads. Finally, the
samples exhibited at least 90% CD19+ cells identified by flow cytometry
(BD Biosciences).
Cell culture and transfection.
MCL cells (Z-138, Mino, REC-1, Jeko-1, and JVM2) were grown in RPMI
1640 medium supplemented with 10% fetal bovine serum (FBS) under 5% CO2
at 37°C. Z-138 and Jeko-1 cells were transiently transfected with USP9X
overexpression or knockdown via Lipofectamine 2000 (Life Technologies
Corp., USA) for 24 h. Moreover, Z-138 and Jeko-1 cells were transiently
transfected with USP9X overexpression and CCND1 knockdown, or USP9X
knockdown and CCND1 overexpression. Additionally, Z-138 and Jeko-1
cells were transiently transfected with USP9X knockdown and SOX11
overexpression.
Plasmids.
For USP9X, CCND1, or SOX11 overexpression, human USP9X, CCND1, or SOX11
cDNA was cloned into the vector pcDNA3.0, respectively. USP9X or CCND1
knockdowns were purchased from JTSBIO (Wuhan, China). The USP9X or
CCND1 shRNA target sequences and scrambled sequences were inserted into
the expression siRNA vector-pRNAH1.1 vector.
Quantitative real-time PCR (qRT-PCR).
Total RNA from peripheral blood mononuclear cells of MCL patients or
MCL cells was extracted using TRIzol (Takara, Dalian, China). Then,
cDNA was synthesized through universal cDNA synthesis kit II (Applied
Biosystems, Foster City, CA, USA). Relative expression of USP9X was
detected and calculated using the 2−ΔΔCT method. Primers were used for this study as follows:
USP9X-F,
5’-CAATGGATAGATCGCTTTATA-3’; USP9X-R, 5’-CTTCTTGCCATGGCCTTAAAT-3’.
CCND1-F, 5’-AGGAAGTTGTGCCAATTTTG−3’; CCND1-R,
5’-AGCAGATGGCCATGCCTTCT-3’. SOX11-F, 5’-AGCAAGAAATGCGGCAAGC-3’;
SOX11-R, 5’-ATCCAGAAACACGCACTTGAC-3’; β-actin-F,
5’-CTACAATGAGCTGCGTGTG-3’; β-actin-R, 5’-TGGGGTGTTGAAGGTCTC-3’.
Western blotting.
Samples were lysed with RIPA lysis buffer. Proteins were extracted and
subjected to SDS-polyacrylamide gels. Membranes were blocked with 5%
skimmed milk and then incubated with primary antibodies, such as USP9X
(1:1000), CDK4 (1:1000), CDK6 (1:1500), P21 (1:1000), PCNA (1:1000),
Bcl-2 (1:1000), Bax (1:1000), Cleaved caspase 3 (1:1000), ANGPT2
(1:1500), FGF1 (1:1000), PDGFA (1:1000), VEGF (1:2000), CCND1 (1:1000),
SOX11 [EPR8192] (1:1000) and β-actin (1:3000) (all from Abcam,
Cambridge, MA, USA) at 4°C overnight. The corresponding HRP-conjugated
secondary antibody was used to cover membranes. Bands were visualized
using enhanced chemiluminescence substrate (Takara, Dalian, China).
Cell viability assay.
The cell viability was measured through a Cell Counting Kit-8 (CCK-8,
Beyotime, Shanghai, China). Cells were seeded into 96 plates, and cell
viability was examined at different periods (0, 24, 48, 72h). The OD
value was detected using an MRX II microplate reader at 450 nm.
BrdU assay.
Cells were fixed with 4% paraformaldehyde and then cut into 5 µm
sections. Sections were deparaffinized and rehydrated and stained with
BrdU. The images were captured under a light microscope.
Cell cycle analysis.
Cells were collected and resuspended through pre-cooled 75% ethanol.
Then, 500ul PBS containing 20ul RNAseA was added to cells for 30 min.
Finally, cells were stained with PI (50 ug/mL) for 25 minutes. Flow
cytometry was used for cell cycle analysis.
Cell apoptosis assay.
Cells were collected and resuspended with 100 μL of binding buffer.
Then, cells were stained with Annexin V-FITC and PI for 15 min.
Finally, cell apoptosis was analyzed through a flow cytometer.
Transwell assay.
For cell invasion analysis, Transwell chambers were added with
Matrigel. For cell migration analysis, Transwell chambers were not
added with Matrigel. Briefly, 4×105
cells were collected and resuspended with an FBS-free medium. Then, 200
μL of suspension was added to the top chamber and 500 μL of medium
supplemented with 10% FBS was added to that lower. The cells were
incubated for 48 h at 37°C, and cells were fixed and stained with 0.1%
crystal violet. The images were observed under a microscope.
Animals. BALB/c nude mice (~4–5 weeks old) were divided into two groups (n=6/group): shNC; shUSP9X-2#. Then, 1 × 106
Z-138 cells with USP9X knockdown (shUSP9X-2#) and control cells (shNC)
were subcutaneously injected into mice. Tumor growth was monitored for
30 days. Last, tumor tissues were collected and photographed when mice
were sacrificed. According to Guide for the Care and Use of Laboratory
Animals and (Yuebei people’s Hospital), all experiments were conducted
in.
Tube formation assay.
Matrigel (50 microliters) was plated in 96-well plates. Human umbilical
vein endothelial cells (HUVECs) were grown on Matrigel with different
treatments and then incubated at 37°C for four h. Finally, tube
formation was observed using microscopy and the branch points were
calculated.
Immunohistochemistry and microvessel density (MVD) analysis.
Tissues were fixed with 4% paraformaldehyde, and then paraffin
embedding was conducted. Paraffin-embedded tissues were cut into
5-µm-thick slides. After blocking with 1% BSA, the samples were
incubated with primary antibodies, including TUNEL (1:1000), Ki67
(1:1500), USP9X (1:1000), CCND1 (1:1000), SOX11 (1:1000), and CD31
(1:1000) (all from Abcam, Cambridge, MA, USA) for 60 min at 37°C and
then covered with goat anti-mouse secondary antibody for 50 min. Cell
nuclei were stained with DAPI. Images were acquired under microscopy.
Additionally, CD31-positive MVD areas were calculated as the sum of
areas of MVD (μm2) evaluated divided by the total size of the core analyzed (μm2) as previously described.[23]
Statistical analysis. Experimental statistical analyses were performed using.
Measurement data were presented as mean ± SD. Two groups were compared with unpaired t-tests,
and comparisons among multiple groups were conducted using ANOVA by
Turkey. P less than 0.5 was considered a statistical significance.
Results
USP9X was increased in PBMCs of MCL patients.
The expression of USP9X, CCND1 and SOX11 in PBMCs of MCL patients was
measured by qRT-PCR and western blotting. The results showed that
USP9X, CCND1, and SOX11 mRNA levels and protein levels were high in
peripheral blood mononuclear cells of MCL patients compared to the
normal sample (Figure 1A, B).
Moreover, a positive correlation between USP9X and CCND1, USP9X and
SOX11, and CCND1 and SOX11 was proved by qRT-PCR, respectively (Figure 1C).
Additionally, qRT-PCR and western blotting demonstrated that USP9X
expression was enhanced in MCL cell lines (Z-138, Mino, REC-1, Jeko-1,
or JVM2) (Figure 1E). These findings suggested that USP9X was increased in PBMCs of MCL patients and MCL cells.
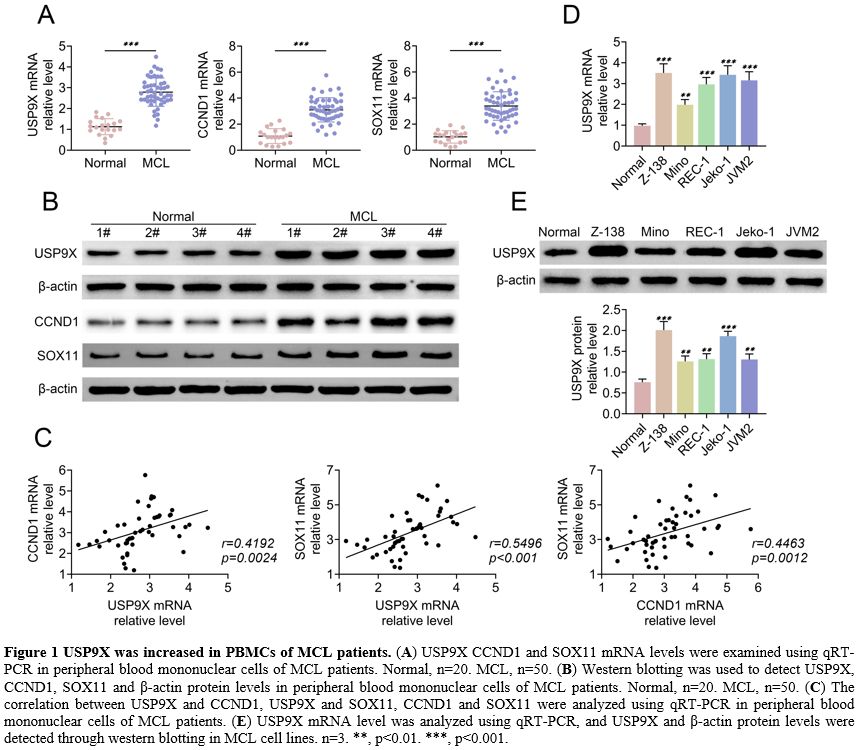 |
Figure 1. USP9X was increased in PBMCs of MCL patients. (A) USP9X
CCND1 and SOX11 mRNA levels were examined using qRT-PCR in peripheral
blood mononuclear cells of MCL patients. Normal, n=20. MCL, n=50. (B) Western
blotting was used to detect USP9X, CCND1, SOX11 and β-actin protein
levels in peripheral blood mononuclear cells of MCL patients. Normal,
n=20. MCL, n=50. (C) The
correlation between USP9X and CCND1, USP9X and SOX11, CCND1 and SOX11
were analyzed using qRT-PCR in peripheral blood mononuclear cells of
MCL patients. (E) USP9X mRNA
level was analyzed using qRT-PCR, and USP9X and β-actin protein levels
were detected through western blotting in MCL cell lines. n=3. **,
p<0.01. ***, p<0.001. |
USP9X promoted proliferation and cell cycle in MCL cells.
The effect of USP9X on proliferation and cell cycle was detected in MCL
cells by inducing either the USP9X overexpression either knockdown.
Western blotting showed that USP9X overexpression increased USP9X
protein level, and USP9X knockdown decreased USP9X protein level in
Z-138 and Jeko-1 (Figure 2A).
CCK-8 assay revealed that cell proliferation was promoted by USP9X
overexpression, whereas it was inhibited by USP9X knockdown in Z-138
and Jeko-1 (Figure 2B). Accordingly, USP9X overexpression elevated BrdU positive cells and USP9X knockdown reduced BrdU positive cells (Figure 2C).
Further, flow cytometry demonstrated that USP9X overexpression
inhibited the G1 stage and promoted the S stage, whereas USP9X
knockdown exerted opposite effects (Figure 2D).
Furthermore, western blotting verified that USP9X overexpression
increased CDK4, CDK6, and PCNA protein levels and decreased P21 protein
level. However, USP9X knockdown reversed these protein levels (Figure 2E). These results indicated that USP9X promoted proliferation and cell cycle in MCL cells.
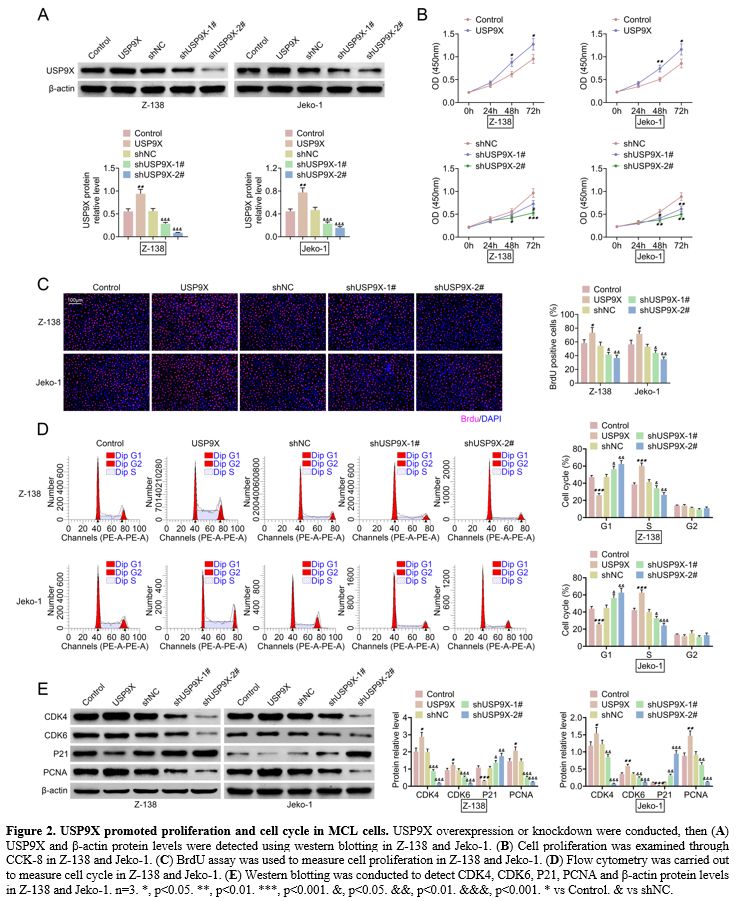 |
Figure
2. USP9X promoted proliferation and cell cycle in MCL cells. USP9X overexpression or knockdown were conducted, then (A) USP9X and β-actin protein levels were detected using western blotting in Z-138 and Jeko-1. (B) Cell proliferation was examined through CCK-8 in Z-138 and Jeko-1. (C) BrdU assay was used to measure cell proliferation in Z-138 and Jeko-1. (D) Flow cytometry was carried out to measure cell cycle in Z-138 and Jeko-1. (E) Western
blotting was conducted to detect CDK4, CDK6, P21, PCNA and β-actin
protein levels in Z-138 and Jeko-1. n=3. *, p<0.05. **, p<0.01.
***, p<0.001. &, p<0.05. &&, p<0.01.
&&&, p<0.001. * vs Control. & vs shNC. |
USP9X inhibited cell apoptosis in MCL cells.
The effect of USP9X on cell apoptosis was explored in MCL cells. The
results from flow cytometry was proved that USP9X overexpression
suppressed cell apoptosis, and USP9X knockdown enhanced cell apoptosis
in MCL cells (Z-138 and Jeko-1) (Figure 3A).
Western blotting demonstrated that USP9X overexpression upregulated
Bcl-2 protein levels and downregulated Bax and Cleaved caspase 3
protein levels. Conversely, USP9X knockdown showed opposite effects on
these protein levels (Figure 3B). The data implied that USP9X inhibited cell apoptosis in MCL cells.
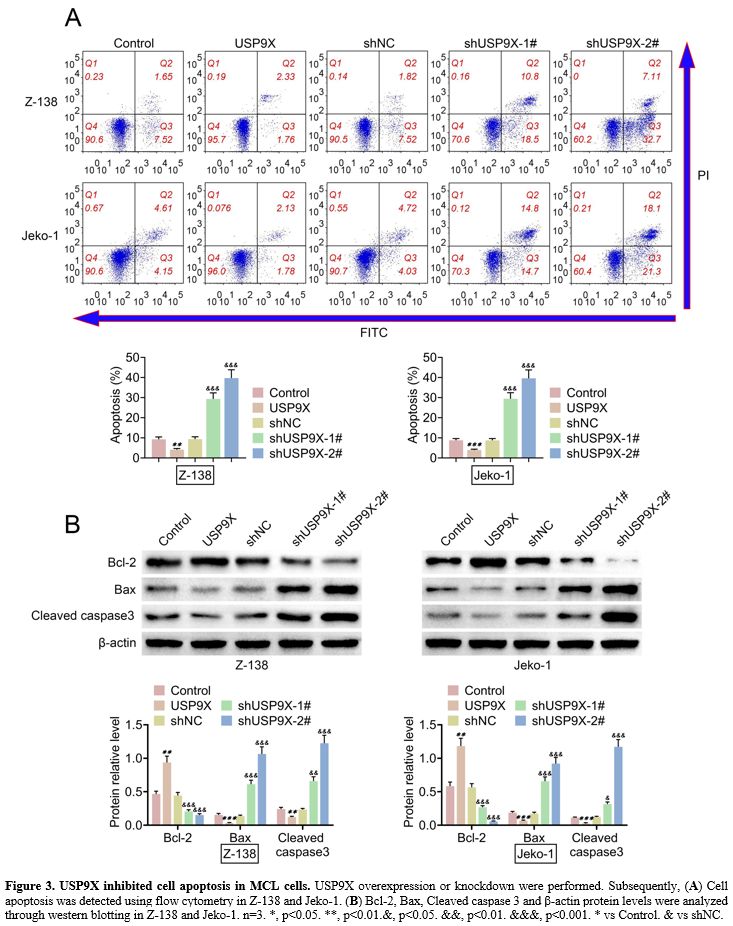 |
Figure 3. USP9X inhibited cell apoptosis in MCL cells. USP9X overexpression or knockdown were performed. Subsequently, (A) Cell apoptosis was detected using flow cytometry in Z-138 and Jeko-1. (B) Bcl-2,
Bax, Cleaved caspase 3 and β-actin protein levels were analyzed through
western blotting in Z-138 and Jeko-1. n=3. *, p<0.05. **,
p<0.01.&, p<0.05. &&, p<0.01. &&&,
p<0.001. * vs Control. & vs shNC. |
USP9X contributed to angiogenesis and promoted cell migration in MCL cells.
The role of USP9X in angiogenesis and cell migration was investigated.
We found that tube formation was increased in HUVECs treated by USP9X
overexpression-positive cell medium (CM), and it was decreased in
HUVECs exposed to USP9X knockdown-positive CM (Figure 4A).
Western blotting verified that USP9X overexpression elevated ANGPT2,
FGF1, PDGFA, and VEGF protein levels, whereas USP9X knockdown repressed
these protein levels in MCL cells (Z-138 and Jeko-1) (Figure 4B).
In addition, cell migration and invasion were promoted by USP9X
overexpression. However, USP9X knockdown inhibited cell migration and
invasion in Z-138 and Jeko-1 (Figure 4C, D).
BrdU revealed that USP9X knockdown decreased cell proliferation. CCND1
overexpression increased USP9X knockdown-caused cell proliferation and
CCND1 T286A further promoted cell proliferation (Supplement Figure 1A).
Flow cytometry proved that the cell cycle inhibited by USP9X knockdown
was enhanced by CCND1 overexpression. In addition, CCND1 T286A further
promoted the cell cycle (Supplement Figure 1B). These results indicated that USP9X contributed to angiogenesis and enabled cells to migrate in MCL tissue.
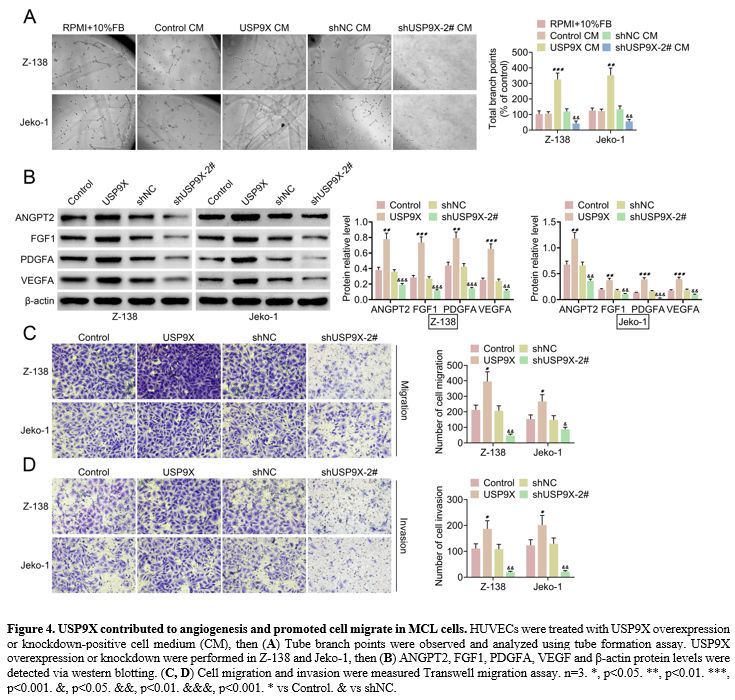 |
Figure 4. USP9X contributed to angiogenesis and promoted cell migrate in MCL cells. HUVECs were treated with USP9X overexpression or knockdown-positive cell medium (CM), then (A) Tube
branch points were observed and analyzed using tube formation assay.
USP9X overexpression or knockdown were performed in Z-138 and Jeko-1,
then (B) ANGPT2, FGF1, PDGFA, VEGF and β-actin protein levels were detected via western blotting. (C, D) Cell
migration and invasion were measured Transwell migration assay. n=3. *,
p<0.05. **, p<0.01. ***, p<0.001. &, p<0.05.
&&, p<0.01. &&&, p<0.001. * vs Control. &
vs shNC. |
USP9X downregulated CCND1-mediated SOX11 expression in MCL cells.
We observed how USP9X could affect CCND1-mediated SOX11 expression in
MCL cells. Western blotting demonstrated that USP9X overexpression
elevated USP9X, CCND1, and SOX11 protein levels, whereas USP9X
knockdown reduced these protein levels in Z-138 and Jeko-1 (Figure 5A).
Furthermore, USP9X overexpression increased USP9X, CCND1, and SOX11
protein levels, and WP1130, a partially selective deubiquitinases (DUB)
inhibitor, decreased USP9X, CCND1, and SOX11 protein levels. However,
USP9X overexpression could promote WP1130-mediated these protein levels
(Figure 5B). Further, western
blotting also showed that CCND1 knockdown inhibited USP9X
overexpression-mediated CCND1 and SOX11 protein levels, and CCND1
overexpression promoted USP9X knockdown-mediated CCND1 and SOX11
protein levels (Figure 5C). These findings suggested that USP9X downregulated CCND1-mediated SOX11 expression in MCL cells.
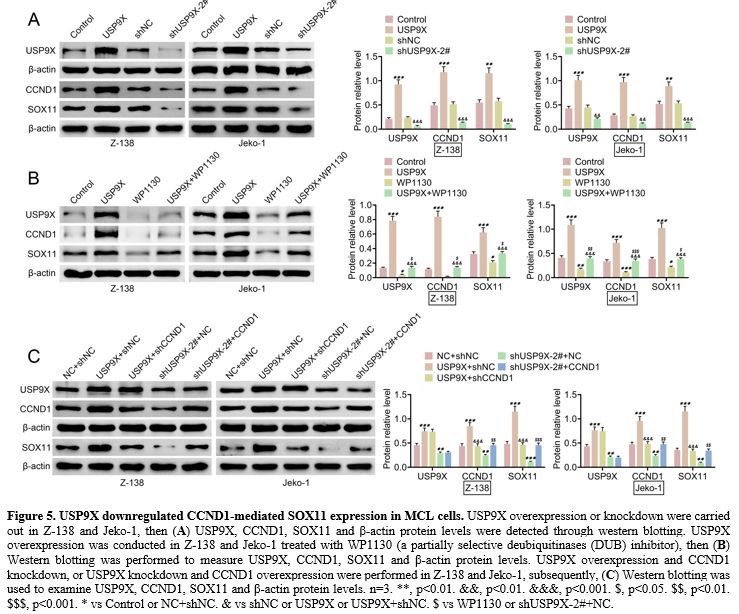 |
Figure 5 USP9X downregulated CCND1-mediated SOX11 expression in MCL cells. USP9X overexpression or knockdown were carried out in Z-138 and Jeko-1, then (A) USP9X,
CCND1, SOX11 and β-actin protein levels were detected through western
blotting. USP9X overexpression was conducted in Z-138 and Jeko-1
treated with WP1130 (a partially selective deubiquitinases (DUB)
inhibitor), then (B) Western
blotting was performed to measure USP9X, CCND1, SOX11 and β-actin
protein levels. USP9X overexpression and CCND1 knockdown, or USP9X
knockdown and CCND1 overexpression were performed in Z-138 and Jeko-1,
subsequently, (C) Western
blotting was used to examine USP9X, CCND1, SOX11 and β-actin protein
levels. n=3. **, p<0.01. &&, p<0.01. &&&,
p<0.001. $, p<0.05. $$, p<0.01. $$$, p<0.001. * vs Control
or NC+shNC. & vs shNC or USP9X or USP9X+shNC. $ vs WP1130 or
shUSP9X-2#+NC. |
SOX11 overexpression reversed USP9X knockdown-mediated angiogenesis in MCL cells. SOX11 overexpression was induced in MCL cells with USP9X knockdown to explore whether SOX11 can regulate
USP9X
knockdown-mediated angiogenesis in MCL cells. Western blotting verified
that SOX11 protein level was inhibited in USP9X knockdown MCL cells and
enhanced in SOX11 overexpression MCL cells. USP9X protein level was
repressed in USP9X knockdown MCL cells, whereas SOX11 overexpression
did not affect USP9X protein level in MCL cells. However, SOX11
overexpression in USP9X knockdown MCL cells suppressed SOX11
overexpression-mediated USP9X and SOX11 protein levels (Figure 6A).
Compared to the USP9X knockdown group, tube formation was enhanced in
HUVECs treated with SOX11 overexpression and USP9X knockdown positive
CM (Figure 6B). Western
blotting demonstrated that USP9X knockdown decreased USP9X protein
level, and SOX11 overexpression showed no effect on USP9X protein level
in MCL cells. However, SOX11 overexpression repressed SOX11
overexpression-mediated USP9X protein levels. Additionally, SOX11
overexpression elevated ANGPT2, FGF1, PDGFA, and VEGF protein levels in
USP9X knockdown MCL cells (Figure 6C).
Further, tube formation was inhibited in HUVECs treated with USP9X
knockdown, whereas CCND1 overexpression enhanced this tube formation (Supplement Figure 2A).
USP9X knockdown repressed ANGPT2, FGF1, PDGFA and VEGF protein levels.
However, CCND1 overexpression showed opposite effects on these protein
levels (Supplement Figure 2B). These results indicated that SOX11 overexpression or CCND1 reversed USP9X knockdown-mediated angiogenesis in MCL cells.
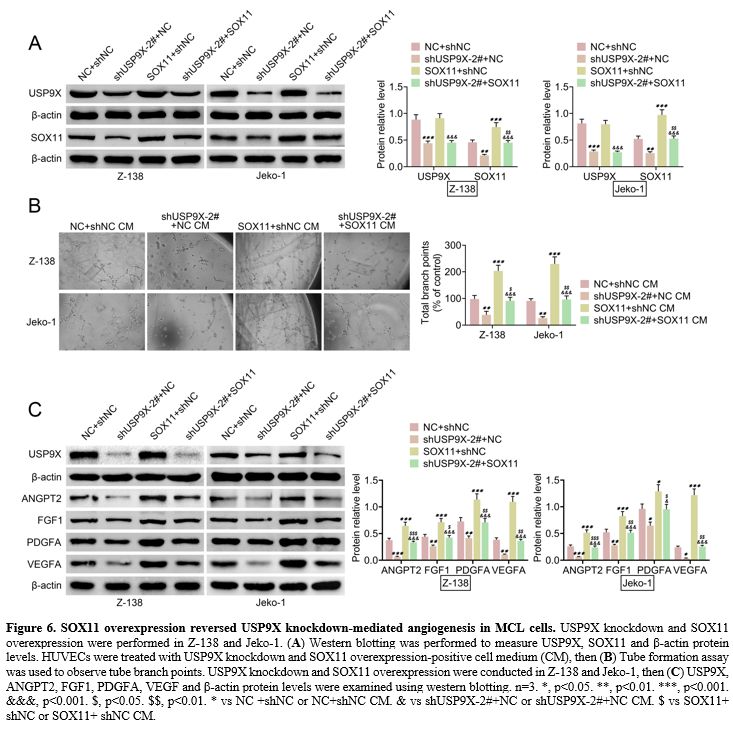 |
Figure 6. SOX11 overexpression reversed USP9X knockdown-mediated angiogenesis in MCL cells. USP9X knockdown and SOX11 overexpression were performed in Z-138 and Jeko-1. (A) Western
blotting was performed to measure USP9X, SOX11 and β-actin protein
levels. HUVECs were treated with USP9X knockdown and SOX11
overexpression-positive cell medium (CM), then (B)
Tube formation assay was used to observe tube branch points. USP9X
knockdown and SOX11 overexpression were conducted in Z-138 and Jeko-1,
then (C) USP9X, ANGPT2, FGF1,
PDGFA, VEGF and β-actin protein levels were examined using western
blotting. n=3. *, p<0.05. **, p<0.01. ***, p<0.001.
&&&, p<0.001. $, p<0.05. $$, p<0.01. * vs NC +shNC
or NC+shNC CM. & vs shUSP9X-2#+NC or shUSP9X-2#+NC CM. $ vs SOX11+
shNC or SOX11+ shNC CM. |
USP9X knockdown inhibited tumor formation in mice. The effect of USP9X knockdown on tumor formation was explored in mice. Figure 7A and B
shows that USP9X knockdown suppressed tumor volume and weight. IHC
showed that USP9X knockdown increased TUNEL expression and decreased
Ki67, USP9X, CCND1, and SOX11 expression in tumor tissues (Figure 7C). Further, MVD revealed that USP9X knockdown repressed CD31 protein level using IHC (Figure 7D). The data suggested that USP9X knockdown inhibited tumor formation in mice.
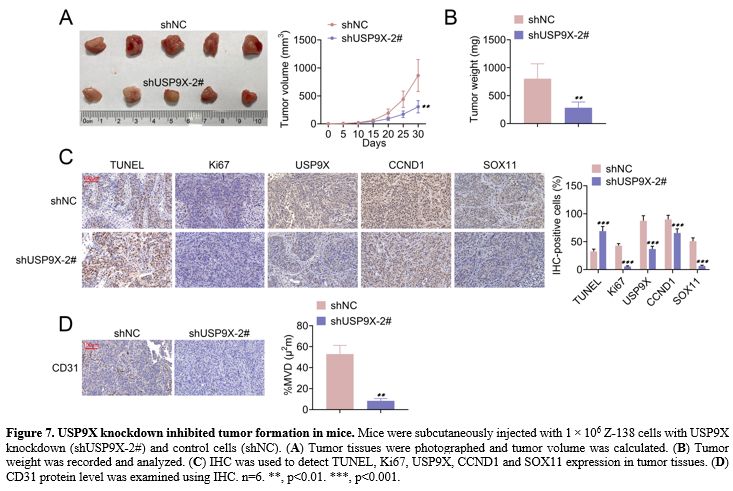 |
Figure 7. USP9X knockdown inhibited tumor formation in mice. Mice were subcutaneously injected with 1 × 106 Z-138 cells with USP9X knockdown (shUSP9X-2#) and control cells (shNC). (A) Tumor tissues were photographed and tumor volume was calculated. (B) Tumor weight was recorded and analyzed. (C) IHC was used to detect TUNEL, Ki67, USP9X, CCND1 and SOX11 expression in tumor tissues. (D) CD31 protein level was examined using IHC. n=6. **, p<0.01. ***, p<0.001. |
Discussion
Here,
USP9X, CCND1, and SOX11 were upregulated in PBMCs of MCL patients.
USP9X level was increased in MCL cells. The positive correlation
between USP9X and CCND1, USP9X and SOX11, and CCND1 and SOX11 were
demonstrated. USP9X was identified to promote proliferation and cell
cycle and inhibited cell apoptosis in MCL cells. Moreover, we found
that USP9X contributed to angiogenesis and increased cell migration in
MCL cells. Further, USP9X downregulated CCND1-mediated SOX11 expression
in MCL cells. SOX11 overexpression was verified to reverse USP9X
knockdown-mediated angiogenesis in MCL cells. In vivo, the results
demonstrated that USP9X knockdown suppressed tumor formation in mice.
Previous
studies have revealed that USP9X could target several cytosolic
proteins and then be involved in regulating multiple cellular
activities, such as cell apoptosis, cell growth, and migration.[24-26] The physical association of USP9X with centrosomes was dependent on the cell cycle and mostly measured in S and G2 phases.[27]
Accordingly, our study demonstrated that USP9X increased proliferation
and cell cycle in MCL cells. Furthermore, cell apoptosis was repressed
by USP9X in MCL cells, evidenced by the upregulation of Bcl-2 protein
level and downregulation of Bax and Cleaved caspase 3 protein levels.
In addition, USP9X was also verified to contribute to angiogenesis and
enhanced cell migration in MCL cells. These findings suggest that USP9X
exerts a key role in MCL cells.
A recent study showed that the
gain-of-function of USP9X took part in increasing CCND1 and decreasing
CDKN1A in breast cancer tissue and cells.[22] CCND1 overexpression was an
early and unifying oncogenic event in MCL. Davis et al. discovered that
CCND1 functioned in the cell cycle in colorectal cancer and MCL.[21]
Chinen et al. revealed that transcript variants of CCND1 were companied
with chromosome 11q13 abnormalities in MCL.[28] Our
study proved that USP9X overexpression promoted CCND1 expression in MCL
cells. USP9X knockdown decreased cell proliferation and cycle. CCND1
overexpression increased USP9X knockdown-caused cell proliferation and
cycle, suggesting that USP9X affects cell proliferation and cycle
through CCND1. However, compared with CCND1 WT, CCND1 T286A further
promoted the USP9X knockdown-mediated cell proliferation and cycle,
indicating that USP9X regulates cell proliferation and cycle by
preventing degradation of CCND1 via the removal of conjugated
ubiquitin. Besides, we found that USP9X overexpression also increased
SOX11 protein levels. Accumulating evidence has shown that SOX11
expression was regulated by CCND1 and STAT3 in MCL.[29] SOX11 has been identified to enhance tumor angiogenesis through PDGFA in MCL.[30]
The relationship between angiogenesis and SOX11 expression has been
revealed, and SOX11 was demonstrated to elevate an angiogenic phenotype
in primary MCL.[31] Interestingly, our research
verified that CCND1 knockdown repressed USP9X overexpression-mediated
SOX11 protein level, suggesting that USP9X downregulated CCND1-mediated
SOX11 expression in MCL cells. USP9X overexpression could promote
WP1130-mediated USP9X, CCND1, and SOX11 protein levels, indicating that
USP9X exerts its function by deubiquitinating Cyclin D1 and/or SOX11.
Additionally, SOX11 overexpression was also found to reverse USP9X
knockdown-mediated angiogenesis in MCL cells. Further analysis showed
that USP9X knockdown could play a suppressive role in tumor formation
in mice. USP9X knockdown reduced tumor volume and weight, inhibited
TUNEL expression, and repressed Ki67, USP9X, CCND1, and SOX11
expression in tumor tissues. The data indicated that USP9X affected
tumor angiogenesis in MCL through regulation of CCND1- mediated SOX11.
However,
the mechanism of USP9X functions in MCL needs to be further explored.
Additionally, the USP9X application in the clinical sample will be
evaluated. Thus, more experiments will be conducted in the near future.
In conclusion, we demonstrated that USP9X contributed to tumor
angiogenesis. Furthermore, USP9X functioned in MCL through
upregulating CCND1- mediated SOX11, which provides a new target for MCL
patients.
Ethics approval
All
procedures performed in studies involving human participants were in
accordance with the standards upheld by the Ethics Committee of Yuebei
people’s Hospital and with those of the 1964 Helsinki Declaration and
its later amendments for ethical research involving human subjects.
The
Ethics Committee approved all animal experiments of Yuebei
people’s Hospital for the use of animals and conducted in accordance
with the National Institutes of Health Laboratory Animal Care and Use
Guidelines.
Statement of Informed Consent
Written
informed consent was obtained from a legally authorized
representative(s) for anonymized patient information to be published in
this article.
Authors’ contributions
Gang
Huang and Jianjun Liao designed the study, supervised the data
collection, Mingli Wang analyzed the data, interpreted the data, and
Yali Huang, Mingjie Tang, and Yanyan Hao prepared the manuscript for
publication and reviewed the draft of the manuscript. All authors have
read and approved the manuscript.
References
- Fakhri B, Kahl B.
Current and emerging treatment
options for mantle cell lymphoma. Therapeutic advances in hematology.
2017; 8(8): 223-34 https://doi.org/10.1177/2040620717719616
PMid:28811872 PMCid:PMC5544150
- Campo E, Rule S. Mantle
cell lymphoma: evolving management strategies. Blood. 2015; 125(1):
48-55 https://doi.org/10.1182/blood-2014-05-521898
PMid:25499451
- Eskelund
CW, Kolstad A, Jerkeman M, Raty R, Laurell A, Eloranta S, Smedby KE,
Husby S, Pedersen LB, Andersen NS, Eriksson M, Kimby E, Bentzen H,
Kuittinen O, Lauritzsen GF, Nilsson-Ehle H, Ralfkiaer E, Ehinger M,
Sundstrom C, Delabie J, Karjalainen-Lindsberg ML, Workman CT, Garde C,
Elonen E, Brown P, Gronbaek K, Geisler CH. 15-year follow-up of the
Second Nordic Mantle Cell Lymphoma trial (MCL2): prolonged remissions
without survival plateau. British Journal of Haematology. 2016; 175(3):
410-8 https://doi.org/10.1111/bjh.14241
PMid:27378674
- Cortelazzo
S, Ponzoni M, Ferreri AJM, Dreyling M. Mantle cell lymphoma. Critical
reviews in oncology/hematology. 2020; 153: 103038 https://doi.org/10.1016/j.critrevonc.2020.103038
PMid:32739830
- Hanel W, Epperla N.
Emerging therapies in mantle cell lymphoma. Journal of Hematology
& Oncology. 2020; 13(1): 79 https://doi.org/10.1186/s13045-020-00914-1
PMid:32552760 PMCid:PMC7302387
- Annese
T, Ingravallo G, Tamma R, De Giorgis M, Maiorano E, Perrone T, Albano
F, Specchia G, Ribatti D. Inflammatory Infiltrate and Angiogenesis in
Mantle Cell Lymphoma. Translational Oncology. 2020; 13(3): 100744 https://doi.org/10.1016/j.tranon.2020.100744
PMid:32120334 PMCid:PMC7052512
- Dreyling
M, Amador V, Callanan M, Jerkeman M, Le Gouill S, Pott C, Rule S, Zaja
F. Update on the molecular pathogenesis and targeted approaches of
mantle cell lymphoma: summary of the 12th
annual conference of the European Mantle Cell Lymphoma Network.
Leukemia & Lymphoma. 2015; 56(4): 866-76 https://doi.org/10.3109/10428194.2014.940584
PMid:25015778
- Wu
C, de Miranda NF, Chen L, Wasik AM, Mansouri L, Jurczak W, Galazka K,
Dlugosz-Danecka M, Machaczka M, Zhang H, Peng R, Morin RD, Rosenquist
R, Sander B, Pan-Hammarstrom Q. Genetic heterogeneity in primary and
relapsed mantle cell lymphomas: Impact of recurrent CARD11 mutations.
Oncotarget. 2016; 7(25): 38180-90 https://doi.org/10.18632/oncotarget.9500
PMid:27224912 PMCid:PMC5122381
- Murtaza
M, Jolly LA, Gecz J, Wood SA. La FAM fatale: USP9X in development and
disease. Cellular and Molecular Life Sciences: CMLS. 2015; 72(11):
2075-89 https://doi.org/10.1007/s00018-015-1851-0
PMid:25672900 PMCid:PMC4427618
- Murray
RZ, Jolly LA, Wood SA. The FAM deubiquitylating enzyme localizes to
multiple points of protein trafficking in epithelia, where it
associates with E-cadherin and beta-catenin. Molecular Biology of the
Cell. 2004; 15(4): 1591-9 https://doi.org/10.1091/mbc.e03-08-0630
PMid:14742711 PMCid:PMC379258
- Theard
D, Labarrade F, Partisani M, Milanini J, Sakagami H, Fon EA, Wood SA,
Franco M, Luton F. USP9x-mediated deubiquitination of EFA6 regulates de
novo tight junction assembly. The EMBO Journal. 2010; 29(9): 1499-509 https://doi.org/10.1038/emboj.2010.46
PMid:20339350 PMCid:PMC2876957
- Liu
L, Yao D, Zhang P, Ding W, Zhang X, Zhang C, Gong S, Zhang Y, Wang J,
Sun T, Ren Z. Deubiquitinase USP9X promotes cell migration, invasion
and inhibits apoptosis of human pancreatic cancer. Oncology Reports.
2017; 38(6): 3531-7 https://doi.org/10.3892/or.2017.6050
PMid:29130109
- Pal
A, Dziubinski M, Di Magliano MP, Simeone DM, Owens S, Thomas D,
Peterson L, Potu H, Talpaz M, Donato NJ. Usp9x Promotes Survival in
Human Pancreatic Cancer and Its Inhibition Suppresses Pancreatic Ductal
Adenocarcinoma In Vivo Tumor Growth. Neoplasia. 2018; 20(2): 152-64 https://doi.org/10.1016/j.neo.2017.11.007
PMid:29248719 PMCid:PMC5735260
- Shen
Q, Reedijk M. Notch Signaling and the Breast Cancer Microenvironment.
Advances in Experimental Medicine and Biology. 2021; 1287: 183-200 https://doi.org/10.1007/978-3-030-55031-8_12
PMid:33034033
- Engel
K, Rudelius M, Slawska J, Jacobs L, Ahangarian Abhari B, Altmann B,
Kurutz J, Rathakrishnan A, Fernandez-Saiz V, Brunner A, Targosz BS,
Loewecke F, Gloeckner CJ, Ueffing M, Fulda S, Pfreundschuh M, Trumper
L, Klapper W, Keller U, Jost PJ, Rosenwald A, Peschel C, Bassermann F.
USP9X stabilizes XIAP to regulate mitotic cell death and
chemoresistance in aggressive B-cell lymphoma. EMBO Molecular Medicine.
2016; 8(8): 851-62 https://doi.org/10.15252/emmm.201506047
PMid:27317434 PMCid:PMC4967940
- Hermeking H. The miR-34
family in cancer and apoptosis. Cell Death and Differentiation. 2010;
17(2): 193-9 https://doi.org/10.1038/cdd.2009.56
PMid:19461653
- Sun
F, Fu H, Liu Q, Tie Y, Zhu J, Xing R, Sun Z, Zheng X. Downregulation of
CCND1 and CDK6 by miR-34a induces cell cycle arrest. FEBS Letters.
2008; 582(10): 1564-8 https://doi.org/10.1016/j.febslet.2008.03.057
PMid:18406353
- Jin
Y, Jin C, Wennerberg J, Hoglund M, Mertens F. Cyclin D1 amplification
in chromosomal band 11q13 is associated with overrepresentation of
3q21-q29 in head and neck carcinomas. International Journal of Cancer.
2002; 98(3): 475-9 https://doi.org/10.1002/ijc.10225
PMid:11920603
- Ahlin
C, Lundgren C, Embretsen-Varro E, Jirstrom K, Blomqvist C, Fjallskog M.
High expression of cyclin D1 is associated to high proliferation rate
and increased risk of mortality in women with ER-positive but not in
ER-negative breast cancers. Breast Cancer Research and Treatment. 2017;
164(3): 667-78 https://doi.org/10.1007/s10549-017-4294-5
PMid:28528450 PMCid:PMC5495873
- Lee
E, Jin D, Lee BB, Kim Y, Han J, Shim YM, Kim DH. Negative effect of
cyclin D1 overexpression on recurrence-free survival in stage II-IIIA
lung adenocarcinoma and its expression modulation by vorinostat in
vitro. BMC cancer. 2015; 15: 982 https://doi.org/10.1186/s12885-015-2001-7
PMid:26681199 PMCid:PMC4683946
- Davis
MI, Pragani R, Fox JT, Shen M, Parmar K, Gaudiano EF, Liu L, Tanega C,
McGee L, Hall MD, McKnight C, Shinn P, Nelson H, Chattopadhyay D,
D'Andrea AD, Auld DS, DeLucas LJ, Li Z, Boxer MB, Simeonov A. Small
Molecule Inhibition of the Ubiquitin-specific Protease USP2
Accelerates cyclin D1 Degradation and Leads to Cell Cycle Arrest in
Colorectal Cancer and Mantle Cell Lymphoma Models. The Journal of
Biological Chemistry. 2016; 291(47): 24628-40 https://doi.org/10.1074/jbc.M116.738567
PMid:27681596 PMCid:PMC5114414
- Li
H, Zheng B. Overexpression of the Ubiquitin-Specific Peptidase 9
X-Linked (USP9X) Gene is Associated with Upregulation of Cyclin D1
(CCND1) and Downregulation of Cyclin-Dependent Inhibitor Kinase 1A
(CDKN1A) in Breast Cancer Tissue and Cell Lines. Medical science
monitor: International Medical Journal of Experimental and Clinical
Research. 2019; 25: 4207-16 https://doi.org/10.12659/MSM.914742
PMid:31169265 PMCid:PMC6568031
- Cardesa-Salzmann
TM, Colomo L, Gutierrez G, Chan WC, Weisenburger D, Climent F,
Gonzalez-Barca E, Mercadal S, Arenillas L, Serrano S, Tubbs R, Delabie
J, Gascoyne RD, Connors JM, Mate JL, Rimsza L, Braziel R, Rosenwald A,
Lenz G, Wright G, Jaffe ES, Staudt L, Jares P, Lopez-Guillermo A, Campo
E. High microvessel density determines a poor outcome in patients with
diffuse large B-cell lymphoma treated with rituximab plus chemotherapy.
Haematologica. 2011; 96(7): 996-1001 https://doi.org/10.3324/haematol.2010.037408
PMid:21546504 PMCid:PMC3128218
- Nagai
H, Noguchi T, Homma K, Katagiri K, Takeda K, Matsuzawa A, Ichijo H.
Ubiquitin-like sequence in ASK1 plays critical roles in the recognition
and stabilization by USP9X and oxidative stress-induced cell death.
Molecular Cell. 2009; 36(5): 805-18 https://doi.org/10.1016/j.molcel.2009.10.016
PMid:20005844
- Homan
CC, Kumar R, Nguyen LS, Haan E, Raymond FL, Abidi F, Raynaud M,
Schwartz CE, Wood SA, Gecz J, Jolly LA. Mutations in USP9X are
associated with X-linked intellectual disability and disrupt neuronal
cell migration and growth. American Journal of Human Genetics. 2014;
94(3): 470-8 https://doi.org/10.1016/j.ajhg.2014.02.004
PMid:24607389 PMCid:PMC3951929
- Dupont
S, Mamidi A, Cordenonsi M, Montagner M, Zacchigna L, Adorno M, Martello
G, Stinchfield MJ, Soligo S, Morsut L, Inui M, Moro S, Modena N,
Argenton F, Newfeld SJ, Piccolo S. FAM/USP9x, a deubiquitinating enzyme
essential for TGFbeta signaling, controls Smad4 monoubiquitination.
Cell. 2009; 136(1): 123-35 https://doi.org/10.1016/j.cell.2008.10.051
PMid:19135894
- Li
X, Song N, Liu L, Liu X, Ding X, Song X, Yang S, Shan L, Zhou X, Su D,
Wang Y, Zhang Q, Cao C, Ma S, Yu N, Yang F, Yao Z, Shang Y, Shi L.
USP9X regulates centrosome duplication and promotes breast
carcinogenesis. Nature Communications. 2017; 8: 14866 https://doi.org/10.1038/ncomms14866
PMid:28361952 PMCid:PMC5380967
- Chinen
Y, Tsukamoto T, Maegawa-Matsui S, Matsumura-Kimoto Y,
Takimoto-Shimomura T, Tanba K, Mizuno Y, Fujibayashi Y, Kuwahara-Ota S,
Shimura Y, Kobayashi T, Horiike S, Taniwaki M, Kuroda J. Tumor-specific
transcript variants of cyclin D1 in mantle cell lymphoma and multiple
myeloma with chromosome 11q13 abnormalities. Experimental Hematology.
2020; 84: 45-53 e1 https://doi.org/10.1016/j.exphem.2020.02.004
PMid:32145384
- Mohanty
A, Sandoval N, Phan A, Nguyen TV, Chen RW, Budde E, Mei M, Popplewell
L, Pham LV, Kwak LW, Weisenburger DD, Rosen ST, Chan WC, Muschen M, Ngo
VN. Regulation of SOX11 expression through CCND1 and STAT3 in mantle
cell lymphoma. Blood. 2019; 133(4): 306-18 https://doi.org/10.1182/blood-2018-05-851667
PMid:30530749 PMCid:PMC6347093
- Palomero
J, Vegliante MC, Rodriguez ML, Eguileor A, Castellano G, Planas-Rigol
E, Jares P, Ribera-Cortada I, Cid MC, Campo E, Amador V. SOX11 promotes
tumor angiogenesis through transcriptional regulation of PDGFA in
mantle cell lymphoma. Blood. 2014; 124(14): 2235-47 https://doi.org/10.1182/blood-2014-04-569566
PMid:25092176
- Petrakis
G, Veloza L, Clot G, Gine E, Gonzalez-Farre B, Navarro A, Bea S,
Martinez A, Lopez-Guillermo A, Amador V, Ribera-Cortada I, Campo E.
Increased tumour angiogenesis in SOX11-positive mantle cell lymphoma.
Histopathology. 2019; 75(5): 704-14 https://doi.org/10.1111/his.13935
PMid:31173643
Supplementary files:
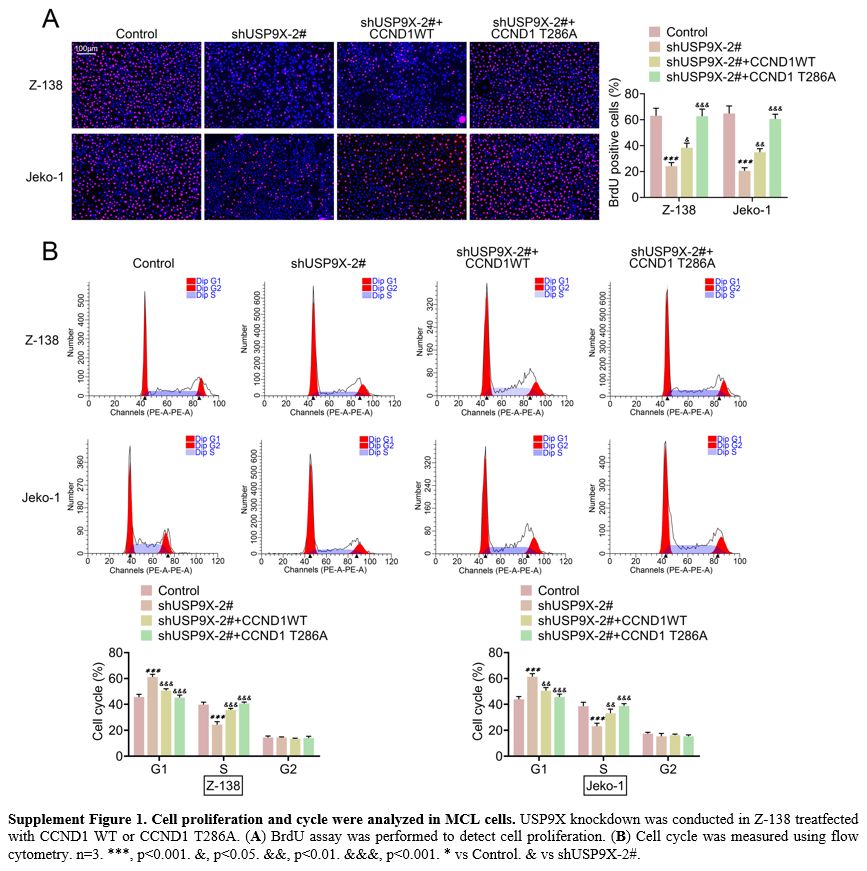 |
Supplement Figure 1. Cell proliferation and cycle were analyzed in MCL cells. USP9X knockdown was conducted in Z-138 treatfected with CCND1 WT or CCND1 T286A. (A) BrdU assay was performed to detect cell proliferation. (B)
Cell cycle was measured using flow cytometry. n=3. ***, p<0.001.
&, p<0.05. &&, p<0.01. &&&, p<0.001. *
vs Control. & vs shUSP9X-2#. |
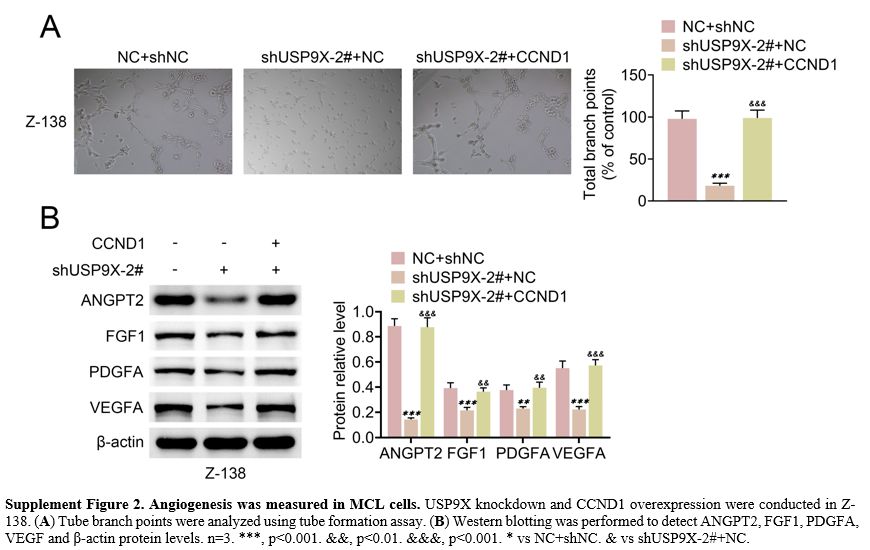 |
Supplement Figure 2. Angiogenesis was measured in MCL cells. USP9X knockdown and CCND1 overexpression were conducted in Z-138. (A) Tube branch points were analyzed using tube formation assay. (B)
Western blotting was performed to detect ANGPT2, FGF1, PDGFA, VEGF and
β-actin protein levels. n=3. ***, p<0.001. &&, p<0.01.
&&&, p<0.001. * vs NC+shNC. & vs shUSP9X-2#+NC. |
[TOP]