Raffaele Palmieri1,2, Giovangiacinto Paterno1, Flavia Mallegni1, Federica Frenza1, Ilenia De Bernardis1, Federico Moretti1, Elisa Meddi1, Maria Ilaria Del Principe1, Luca Maurillo1, Adriano Venditti1 and Francesco Buccisano1.
1 Department of Biomedicine and Prevention, University of Rome Tor Vergata, Rome, Italy
2 Clinical Research Division, Fred Hutchinson Cancer Center, Seattle (WA), USA.
Published: September 1, 2023
Received: July 18, 2023
Accepted: August 11, 2023
Mediterr J Hematol Infect Dis 2023, 15(1): e2023051 DOI
10.4084/MJHID.2023.051
This is an Open Access article distributed
under the terms of the Creative Commons Attribution License
(https://creativecommons.org/licenses/by-nc/4.0),
which permits unrestricted use, distribution, and reproduction in any
medium, provided the original work is properly cited.
|
Abstract
Therapy-related myeloid neoplasms
(t-MNs) encompass a specific sub-group of myeloid malignancies arising
after exposure to radio/cytotoxic agents for the treatment of unrelated
diseases. Such malignancies present unique features, including
advanced age, high comorbidities burden, and unfavorable genetic
profiles. All these features justify the need for a specific diagnostic
work-up and dedicated treatment algorithms. However, as new
classification systems recognize the unique clinical characteristics
exhibited by t-MN patients, how to assess fitness status in this
clinical setting is largely unexplored. Optimizing fitness assessment
would be crucial in the management of t-MN patients, considering that
factors usually contributing to a worse or better outcome (like age,
comorbidities, and treatment history) are patient-specific. In the
absence of specific tools for fitness assessment in this peculiar
category of AML, the aim of this review is to describe all those
factors related to patient, treatment, and disease that allow planning
treatments with an optimal risk/benefit ratio.
|
Introduction
The
specificity of myeloid neoplasms that arise following exposure to
cytotoxic chemotherapy, ionizing radiotherapy, and/or immunosuppressive
therapy for an unrelated antecedent disease is recognized as a specific
sub-group, commonly referred to as therapy-related myeloid neoplasms
(t-MNs).[1,2] Nevertheless, according to the
recent version of World Health Organization (WHO) and International
Consensus Classification (ICC) of myeloid neoplasms, characteristics
related to patients and disease, rather than clinical history, seem to
account for the differences observed between therapy-related and de novo
neoplasms. As a result, both classifications now consider
therapy-relatedness as a “disease qualifier” rather than a disease
defining entity.[3,4]
The approach to this
subgroup of acute myeloid leukemias (t-AML) and myelodysplastic
syndromes (t-MDS) presents several challenges. In the large majority of
cases, t-MNs typically present with unfavorable features, such as
peripheral blood cytopenias and high-risk genetic and cytogenetic
profile.[5] Furthermore, from a clinical point of
view, the previous exposure to anticancer treatments may significantly
affect fitness for antileukemic treatment. Consequently, even for young
fit patients, 5-year overall survival hardly reaches 20-25%.[6] Taken together, all these features require a specific diagnostic work-up and dedicated treatment algorithms.[7]
The
purpose of this review is to explore the need of a specific approach to
the fitness evaluation of t-AML patients and to pinpoint possible
solutions to select treatments, included allogeneic stem cell
transplantation (ASCT), with the optimal risk/benefit ratio.
Current Status of t-MNs Classification
Recently,
the fifth version of the WHO Classification of Hematolymphoid Tumors
has slightly modified the definition of t-MNs, speculating that
pre-existing clonal hematopoiesis may play a role as a risk factor for
the expansion of pre-existing (non-neoplastic) clones. This hypothesis
is supported by the fact that only a minority of patients receiving
mutagenic agents will develop t-MNs in their lifetime. As an additional
point, most of such cases are associated with recurrent cytogenetic and
molecular signatures, hinting that specific genetic lesions may emerge
due to selection pressures of cytotoxic therapy agents in an altered
bone marrow environment.[3] In line with this, the ICC
of myeloid neoplasms and acute leukemias underlines that, although it
remains important to recognize the therapy-relatedness of MNs, the
first priority is to classify the disease according to its morphologic
and genetic characteristics.[4]
Since its first
recognition as a discrete entity, the criteria to be fulfilled for the
definition of t-MNs have changed over time. Although prior exposure to
radiotherapy or chemotherapy has always been considered a prerequisite
for t-MN development, the list of “trigger drugs” and the latency
between exposition to each treatment and disease manifestation have
been periodically updated.[8,9,2]
This evolution reflects not only the constant improvement in the
understating of the mutagenic mechanisms of chemotherapy but also the
need to include novel agents in the debate.
The main causative
agents involved in the development of t-MNs include alkylating agents,
ionizing radiations, and topoisomerase II inhibitors.[3]
Patients previously exposed to alkylating agents or ionizing radiation
tend to present with MDS after a median of 4-10 years from treatment
exposure.[10,11] Many of these patients may
eventually progress to AML, presenting with a loss of genetic materials
(such as deletions involving chromosomes 5, 7, and 17), complex
karyotype, and TP53 deletions.[12] The other way
around, patients receiving topoisomerase II inhibitors develop t-MNs
with a significantly shorter latency from exposure (1 to 5 years).
Balanced chromosomal translocations, including t(16;16), t(8;21),
t(9;22) and MLL involving chromosome band 11q23, are frequently
observed in this second group.[13] In recent years, a
third group of drugs targeting enzymes involved in DNA repair
mechanisms (inhibitors of the enzyme poly ADP ribose polymerase, also
called PARP inhibitors) were added to the list of agents with a
documented influence on t-MN development.[14]
Patients receiving PARP inhibitors are at higher risk of t-MNs with a
two-year latency, especially when administered in association with
alkylating agents.[15] Accordingly, exposure to such agents was added as a qualifying criterion for t-MNs in the latest WHO classification.[3]
Regarding
t-MDS, despite the diagnosis and the consequent therapy being
established following the same criteria adopted for their de novo
counterpart, these diseases exhibit substantial genetic/cytogenetic and
clinical differences. Accordingly, a future updated classification
should consider this issue, possibly identifying t-MDS as a distinct
sub-group.[16]
Although the majority of t-MNs
are associated with high-risk defining genetic lesions (such as TP53
mutations), some cases may present with a de novo molecular signature, such as isolated NPM1 mutations, Acute Promyelocytic Leukemia, and core-binding factor leukemias.[17,18]
Following the general rule according to which post-cytotoxic therapy
designation is based on the medical history, these cases are currently
classified as t-MNs. However, as t-MN patients characterized by these
specific genetic/cytogenetic signatures seem to do well with
conventional intensive chemotherapy, whether they should be considered
or not as “low risk” (and treated accordingly) is still a matter of
debate.[17-19]
Specific Consideration for Fitness in t-AML Patients
Clinical
presentation of t-MNs can be extremely heterogeneous and heavily
influenced by several factors that must be considered during treatment
planning.[20] Patient-related characteristics, such
as advanced age, lower performance status, a high number of concomitant
comorbidities, and past medical history (including the type of
antecedent cancer and treatment received) have historically qualified
many t-MNs patients as “unfit” and therefore less likely to be included
in curative-intended protocols.[21] The scenario is
further complicated by the fact that more effective and
better-tolerated treatment alternatives are limited and still under
investigation.[22]
The price all patients pay
when receiving chemo/radiotherapy is a reduction in the functional
reserve of all organs and tissues involved (Figure 1).[23]
These effects can be either acute and self-limiting or chronic and may
worsen in the case of the administration of additional cytotoxic
agents.[24] In this view, an accurate anamnesis is a
fundamental step to calculate the total dose of drugs known to have a
maximum cumulative dose. Anthracyclines, for instance, are
antineoplastic agents with proven efficacy in a broad variety of
neoplastic conditions (including breast, ovarian, bladder, and lung
cancers, Wilms’ tumor, both Hodgkin’s and Non-Hodgkin’s Lymphomas,
acute leukemias) and whose administration has been associated with
dose-dependent cardiotoxicity (> 450 mg/m2 over a lifetime).[25] Similarly, mitoxantrone at doses of more than 140 mg/m2 can cause congestive heart failure and induce cardiomyopathy.[26]
Older patients, especially those with concomitant cardiac
comorbidities, are at higher risk of anthracyclines-induced
cardiomyopathy even when not reaching the maximum cumulative dose. When
performing pre-treatment cardiac testing, a decrease in left
ventricular ejection fraction to less than 45% may suggest
anthracycline-induced cardiotoxicity.[26] Therefore,
in patients deemed at risk of developing acute cardiac complications on
re-administration of anthracyclines, avoidance of such agents may be a
reasonable precaution.
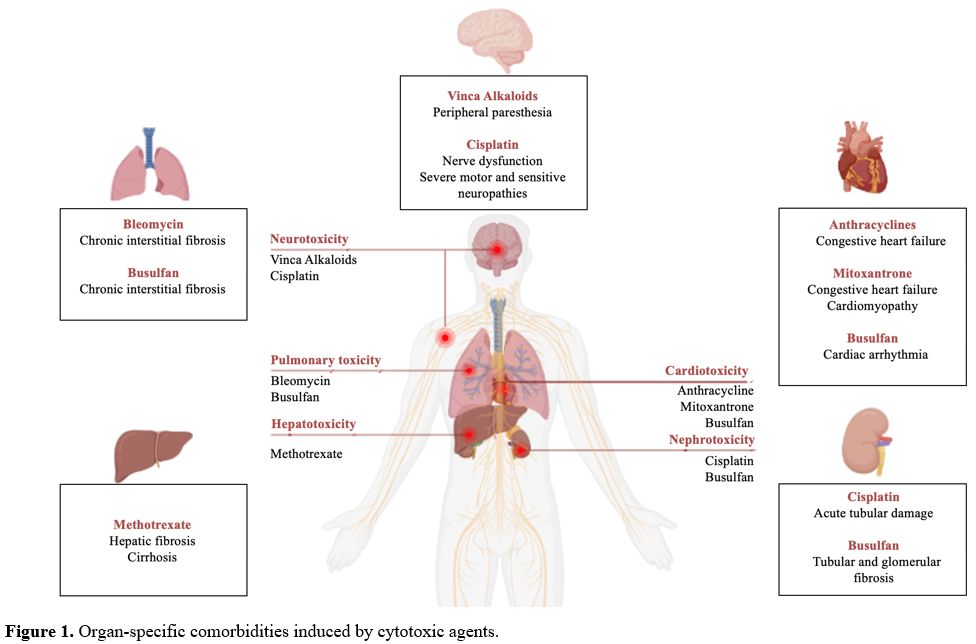 |
- Figure 1. Organ-specific comorbidities induced by cytotoxic agents.
|
Along
with cardiovascular toxicities, there are specific cytotoxic agents
able to induce a dose-dependent irreversible pulmonary failure.
Bleomycin is a drug used to treat several malignancies, including
head-neck tumors, testicular and ovarian cancers, and lymphomas.
Pulmonary diseases induced by such agent are generally observed around
the maximum cumulative dose of 400-450 mg and mainly consist of chronic
interstitial fibrosis.[27] In patients with t-MNs and
prior exposure to such medication, periodical pulmonary function
testing should be performed to treat those who develop pulmonary
complications in a timely manner. As pulmonary abnormalities seem play
a major role in the definition of fitness,[28]
periodical pulmonary function testing could be an option in patients
with t-MNs and prior exposure to Bleomycin. In case of pulmonary
disfunction, such an approach could help treat those patients who
develop pulmonary complications in a timely manner.
Several agents
commonly adopted in a wide variety of tumors may have an influence on
renal function. Among these, cisplatin and its derivates are used to
treat different types of cancers, including testicular, ovarian,
bladder, head-neck, lung, and cervical. A non-negligible proportion of
patients (roughly 30%) receiving cisplatin will develop nephrotoxicity
with a single dose of 2 mg/kg or 50-75 mg/m2, especially when not adequately hydrated.[29]
Acute kidney damage can present within a single day from a single dose
of cisplatin, and patients may lose up to 12.5% of their renal function
after receiving this medication.[30] Subjects
previously exposed to cisplatin should be vigorously hydrated in
conditions at high risk of acute tubular damage, such as hyper
leucocytic onset, and to prevent tumor lysis syndrome in all cases.
Iatrogenic
hepatic damage can be observed in patients submitted to specific agents
for the treatment of both neoplastic and non-neoplastic conditions.
Methotrexate is frequently prescribed at high doses in many cancers
(such as non-Hodgkin’s lymphomas) and at lower doses in autoimmune
disorders (including systemic lupus erythematosus and rheumatoid
arthritis). When administered in patients already taking putative
hepatotoxic drugs or in those with other liver dysfunctions (alcohol
liver disease or metabolic syndrome), methotrexate can cause hepatic
fibrosis and cirrhosis.[31] Routine function testing,
as well as periodic imaging, can be helpful in the identification of
gross liver diseases. Furthermore, in patients with clinical and/or
radiological evidence of chronic liver disease, transient elastography
may be an option to assess the severity of the hepatic dysfunction and
to optimize (or even avoid) the use of hepatotoxic anti-cancer drugs.[32]
Neurotoxicity
represents a common side-effect of many anti-cancer therapies. Vinca
alkaloids and cisplatin can cause central, peripheral, and even
autonomic nervous system toxicities. Usually starting as peripheral
paresthesia, nerve dysfunction can progress up to severe motor and
sensitive neuropathies.[33] Although uncommonly
life-threatening, such conditions can have a relevant impact on daily
activities and quality of life, hence deserving of proper management.
Whichever
the cytotoxic agent delivered in the past, whether specific organ
dysfunctions may be evident or not, extreme caution is needed when
considering the eligibility/ineligibility of each patient with t-MNs to
a given therapy. This may be particularly relevant when facing the
opportunity to administer cytotoxic agents that have caused a
significant reduction in organ functional reserve. An additional unmet
need is represented by those patients who are diagnosed with
concomitant t-MN and recurrent solid tumor. Since we still lack robust
evidence on the actual feasibility of a simultaneous approach and how
these patients may truly benefit from it, such circumstance commonly
justifies referral to palliative care for most cases.[34]
An
additional factor to be considered during treatment planning is the
type of antecedent solid tumor. Although this information may not be
crucial to decide treatment intensity, t-MNs following specific cancers
(such as lymphoproliferative disorders) seem to be characterized by
significantly shorter survival than others (such as breast cancers),
whose outcome resembles that of de novo AML/MDS.[35,36]
Even
though there are several validated scores for the definition of
fitness/unfitness in patients with de novo myeloid neoplasms, all these
tools fail to include information on how to modulate treatment
intensity in t-MPs.[20] Consequently, fitness
assessment in t-MNs patients mainly relies on a case-by-case evaluation
that should take into account factors related to patient, treatment and
disease. (Figure 2) In the absence of dedicated tools, further investigation is expected to address this issue, in the near future.
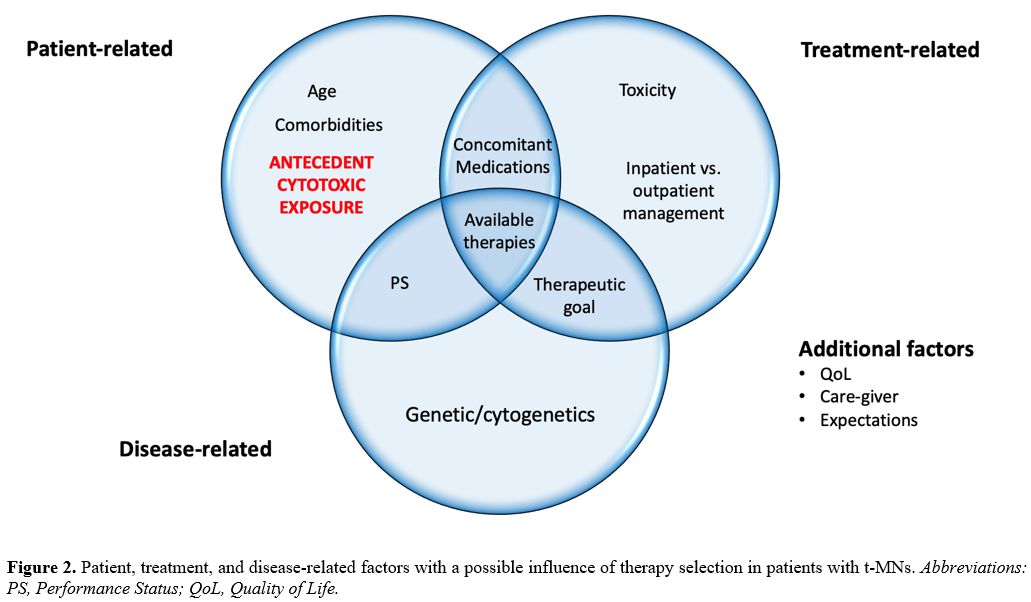 |
- Figure 2. Patient,
treatment, and disease-related factors with a possible influence of
therapy selection in patients with t-MNs. Abbreviations: PS, Performance Status; QoL, Quality of Life.
|
Hematopoietic Stem Cell Transplantation in t-MNs
The
criteria to define the eligibility for hematopoietic stem cell
transplantation (HCT) have been periodically updated. As a result of
this constant improvement, more and more fit patients with blood
cancers are referred to HCT every year. Patients with t-MNs represent
the paradigm of high-risk diseases for whom HCT may represent the only
chance for cure. Nevertheless, these patients are also at higher risk
of experiencing HCT-related early and late complications. Although the
long-term curative potential of HCT in such diseases is unquestioned,
for some patients, the risks may outweigh the benefits. The evidence
that even a remote history of an unspecified solid tumor may harm HCT
long-term outcome has prompted the inclusion of the anamnestic criteria
into scores commonly adopted to assess transplant eligibility.[37]
Chemo-radiotherapy
that must be delivered as a pre-transplant conditioning regimen may
have an additional impact on the residual functional reserve of
specific organs and tissues. Agents like busulfan, which has
historically represented the cornerstone of many conditioning regimens,
can cause cardiac, renal, and pulmonary side effects shortly after
infusion.[38] This evidence underlines the importance
of modulating conditioning strategies in patients who already show
signs or symptoms of organ insufficiencies before HCT. If possible,
reduced-intensity conditioning (RIC) or non-myeloablative (NMA)
regimens may be a reasonable option in this category.
In t-MN patients who are considered fit at the time of diagnosis, HCT is not always feasible.[21]
Not uncommonly, the odds of exacerbating pre-existing organ frailties,
even when delivering RIC or NMA, represent a significant
contraindication to HCT. Unfortunately, this eventuality can’t be
predicted at the time of t-MN diagnosis and many patients witness the
interruption of their curative program after developing severe side
effects during induction or consolidation therapy. Such complications
are almost always unforeseeable, with a dramatic impact not only on
life expectancy but also on quality of life.[39]
Another
issue to be considered during the pre-HCT workup is that
immunosuppressive medications, usually administered as
graft-versus-host disease prophylaxis, may increase the probability of
relapse of pre-existing tumors.[40] In some
institutions, potential transplant recipients who have a history of a
solid tumor are considered eligible for HCT if the probability of
recurrence of the solid tumor is estimated to be <20% over 5 years
at the time of the pre-transplant evaluation.[40]
These criteria were initially proposed for kidney transplant candidates
but are applicable to HCT too. Although based on retrospective studies,
the risk of solid tumor recurrence after HCT in patients with low
localized (e.g., Stage I-II)/under control disease may justify
transplant delivery. The risk of relapse in this setting is
considerably outweighed by the benefit if we consider that a
hematologic malignancy requiring HCT usually cannot wait for several
months or years while being monitored for recurrent solid tumors.[40,41]
As a possible solution for patients whose prior tumor is under control,
a multi-disciplinary pre-transplant evaluation would afford more
patients the option of HCT. Furthermore, a close collaboration between
the cancer specialist and the transplant team would be essential to
optimize the treatment strategy of those patients who experience a
tumor relapse after HCT. Likewise, for patients at higher risk of solid
tumor relapse, delivering adjuvant or even post-transplant maintenance
therapy could represent an additional solution to reduce the risk of
solid tumor recurrence, thus affording more patients the option of HCT.[42]
In this view, how to update cancer specific HCT eligibility criteria
and how to personalize post-transplant follow up in this group of
patients represents an urgent need.
Discussion
The
recent classifications consider therapy-relatedness as a qualifier of
AML rather than a specific category of disease. Nevertheless, since
t-MNs usually present with peculiar clinical and biological
characteristics, dedicated therapeutic algorithms are necessary.
The
available tools to assess eligibility for intensive or non-intensive
therapies are not specifically designed for t-MNs. Anamnestic features
and type of prior cytotoxic exposure are case-specific and may have
determined long-term effects that deserve to be considered during
treatment planning. In line with the change observed for classification
systems, t-MN cases should not simply be identified as being
“high-risk” patients and treated or not treated accordingly but deserve
individualized pre-treatment evaluations.
In fact, some patients
may present with multiple comorbidities or end-stage organ failures
that may have been induced by prior cytotoxic agents. Alternatively,
t-MNs may emerge while a patient is already receiving active treatment
for the relapse of the antecedent neoplasm. Furthermore, the presence
of t-MNs frequently excludes patients from innovative clinical trials.[42]
Defining
fitness status in this clinical setting currently relies on the
application of the same scores that have been designed and validated in
patients with de novo myeloid
neoplasms. Persevering with such an approach may fail to offer
appropriate estimates of the applicability of emerging treatment
strategies. In a future perspective, designing dedicated scores is
warranted and may help optimize managing such “hard-to-treat” diseases.
References
- Fianchi L, Criscuolo M, Fabiani E, et al.
Therapy-related myeloid neoplasms: Clinical perspectives. Onco Targets
Ther. 2018;11:5909-5915. doi:10.2147/OTT.S101333 https://doi.org/10.2147/OTT.S101333 PMid:30271175 PMCid:PMC6149829
- Arber
DA, Orazi A, Hasserjian R, et al. The 2016 revision to the World Health
Organization classification of myeloid neoplasms and acute leukemia.
Blood. 2016;127(20):2391-2405. doi:10.1182/blood-2016-03-643544 https://doi.org/10.1182/blood-2016-03-643544 PMid:27069254
- Khoury
JD, Solary E, Abla O, et al. The 5th edition of the World Health
Organization Classification of Haematolymphoid Tumours: Myeloid and
Histiocytic/Dendritic Neoplasms. Leukemia. 2022;36(7):1703-1719.
doi:10.1038/s41375-022-01613-1 https://doi.org/10.1038/s41375-022-01613-1 PMid:35732831 PMCid:PMC9252913
- Arber
DA, Orazi A, Hasserjian RP, et al. International Consensus
Classification of Myeloid Neoplasms and Acute Leukemias: integrating
morphologic, clinical, and genomic data. Blood. 2022;140(11):1200-1228.
doi:10.1182/blood.2022015850 https://doi.org/10.1182/blood.2022015850 PMid:35767897
- Voso
MT, Falconi G, Fabiani E. What's new in the pathogenesis and treatment
of therapy-related myeloid neoplasms. Blood. 2021;138(9):749-757.
doi:10.1182/blood.2021010764 https://doi.org/10.1182/blood.2021010764 PMid:33876223
- Litzow
MR, Tarima S, Pérez WS, et al. Allogeneic transplantation for
therapy-related myelodysplastic syndrome and acute myeloid leukemia.
Blood. 2010;115(9):1850-1857. doi:10.1182/blood-2009-10-249128 https://doi.org/10.1182/blood-2009-10-249128 PMid:20032503 PMCid:PMC2832815
- Döhner
H, Wei AH, Appelbaum FR, et al. Diagnosis and management of AML in
adults: 2022 recommendations from an international expert panel on
behalf of the ELN. Blood. 2022;140(12):1345-1377.
doi:10.1182/blood.2022016867 https://doi.org/10.1182/blood.2022016867 PMid:35797463
- Vardiman
JW, Harris NL, Brunning RD. The World Health Organization (WHO)
classification of the myeloid neoplasms. Blood. 2002;100(7):2292-2302.
doi:10.1182/blood-2002-04-1199 https://doi.org/10.1182/blood-2002-04-1199 PMid:12239137
- Vardiman
JW, Thiele J, Arber DA, et al. The 2008 revision of the World Health
Organization (WHO) classification of myeloid neoplasms and acute
leukemia: Rationale and important changes. Blood. 2009;114(5):937-951.
doi:10.1182/blood-2009-03-209262 https://doi.org/10.1182/blood-2009-03-209262 PMid:19357394
- Tiruneh
T, Enawgaw B, Shiferaw E. Genetic Pathway in the Pathogenesis of
Therapy-Related Myeloid Neoplasms: A Literature Review. Oncol Ther.
2020;8(1):45-57. doi:10.1007/s40487-020-00111-7 https://doi.org/10.1007/s40487-020-00111-7 PMid:32700075 PMCid:PMC7360004
- Joannides
M, Grimwade D. Molecular biology of therapy-related leukaemias.
Clinical and Translational Oncology. 2010;12(1):8-14.
doi:10.1007/s12094-010-0460-5 https://doi.org/10.1007/s12094-010-0460-5 PMid:20080465
- Qian
Z, Joslin JM, Tennant TR, et al. Cytogenetic and genetic pathways in
therapy-related acute myeloid leukemia. Chem Biol Interact.
2010;184(1-2):50-57. doi:10.1016/j.cbi.2009.11.025 https://doi.org/10.1016/j.cbi.2009.11.025 PMid:19958752 PMCid:PMC4642715
- Economides
MP, McCue D, Borthakur G, Pemmaraju N. Topoisomerase II inhibitors in
AML: past, present, and future. Expert Opin Pharmacother.
2019;20(13):1637-1644. doi:10.1080/14656566.2019.1621292 https://doi.org/10.1080/14656566.2019.1621292 PMid:31136213
- Csizmar
CM, Saliba AN, Swisher EM, Kaufmann SH. PARP Inhibitors and Myeloid
Neoplasms: A Double-Edged Sword. Cancers (Basel). 2021;13(24):1-34.
doi:10.3390/cancers13246385 https://doi.org/10.3390/cancers13246385 PMid:34945003 PMCid:PMC8699275
- Morice
PM, Leary A, Dolladille C, et al. Myelodysplastic syndrome and acute
myeloid leukaemia in patients treated with PARP inhibitors: a safety
meta-analysis of randomised controlled trials and a retrospective study
of the WHO pharmacovigilance database. Lancet Haematol.
2021;8(2):e122-e134. doi:10.1016/S2352-3026(20)30360-4 https://doi.org/10.1016/S2352-3026(20)30360-4 PMid:33347814
- Leone G, Fabiani E, Voso MT. De Novo and Therapy-Related Myelodysplastic Syndromes: Analogies and Differences. Mediterr J Hematol Infect Dis. 2022; 14(1): e2022030. Published online 2022 May 1. doi: 10.4084/MJHID.2022.030 https://doi.org/10.4084/MJHID.2022.030 PMid:35615324 PMCid:PMC9083943
- Othman
J, Meggendorfer M, Tiacci E, et al. Overlapping features of
therapy-related and de novo NPM1 -mutated AML. Blood.
2023;141(15):1846-1857. doi:10.1182/blood.2022018108 https://doi.org/10.1182/blood.2022018108 PMid:36508705
- Larson RA, Le Beau MM. Prognosis
and Therapy When Acute Promyelocytic Leukemia and Other "Good Risk"
Acute Myeloid Leukemias Occur as a Therapy-Related Myeloid Neoplasm. Mediterr J Hematol Infect Dis. 2011; 3(1): e2011032. Published online 2011 Jul 8. doi: 10.4084/MJHID.2011.032 https://doi.org/10.4084/mjhid.2011.032 PMid:21869918 PMCid:PMC3152454
- Pulsoni
A, Pagano L, Lo Coco F, et al. Clinicobiological features and outcome
of acute promyelocytic leukemia occurring as a second tumor: the GIMEMA
experience. Blood. 2002 Sep 15;100(6):1972-6. doi:
10.1182/blood-2001-12-0312. https://doi.org/10.1182/blood-2001-12-0312 PMid:12200354
- Palmieri
R, Paterno G, De Bellis E, et al. Therapeutic choice in older patients
with acute myeloid leukemia: A matter of fitness. Cancers (Basel).
2020;12(1):1-19. doi:10.3390/cancers12010120 https://doi.org/10.3390/cancers12010120 PMid:31906489 PMCid:PMC7016986
- Kayser
S, Döhner K, Krauter J, et al. The impact of therapy-related acute
myeloid leukemia (AML) on outcome in 2853 adult patients with newly
diagnosed AML. Blood. 2011;117(7):2137-2145.
doi:10.1182/blood-2010-08-301713 https://doi.org/10.1182/blood-2010-08-301713 PMid:21127174
- Strickland
SA, Vey N. Diagnosis and treatment of therapy-related acute myeloid
leukemia. Crit Rev Oncol Hematol. 2022;171:103607.
doi:10.1016/j.critrevonc.2022.103607 https://doi.org/10.1016/j.critrevonc.2022.103607 PMid:35101585
- Zeien
J, Qiu W, Triay M, et al. Clinical implications of chemotherapeutic
agent organ toxicity on perioperative care. Biomedicine and
Pharmacotherapy. 2022;146(September 2021):112503.
doi:10.1016/j.biopha.2021.112503 https://doi.org/10.1016/j.biopha.2021.112503 PMid:34922113
- Miller
KD, Nogueira L, Mariotto AB, et al. Cancer treatment and survivorship
statistics, 2019. CA Cancer J Clin. 2019;69(5):363-385.
doi:10.3322/caac.21565 https://doi.org/10.3322/caac.21565 PMid:31184787
- Neuendorff
NR, Loh KP, Mims AS, et al. Anthracycline-related cardiotoxicity in
older patients with acute myeloid leukemia: A Young SIOG review paper.
Blood Adv. 2020;4(4):762-775. doi:10.1182/bloodadvances.2019000955 https://doi.org/10.1182/bloodadvances.2019000955 PMid:32097461 PMCid:PMC7042993
- Ganz
WI, Sridhar KS, Ganz SS, Gonzalez R, Chakko S, Serafini A. Review of
Tests for Monitoring Doxorubicin-lnduced Cardiomyopathy. Oncology
(Switzerland). 1996;53(6):461-470. doi:10.1159/000227621 https://doi.org/10.1159/000227621 PMid:8960141
- Liu T, De Los Santos FG, Phan SH. The Bleomycin Model of Pulmonary Fibrosis. In: ; 2017:27-42. doi:10.1007/978-1-4939-7113-8_2 https://doi.org/10.1007/978-1-4939-7113-8_2 PMid:28836192
- Palmieri
R, Othus M, Cheng SG, et al. Pulmonary function testing for fitness
assessment in asymptomatic adults with newly diagnosed acute myeloid
leukemia. Haematologica. 2022 Nov 1;107(11):2752-2755. doi:
10.3324/haematol.2022.281445 https://doi.org/10.3324/haematol.2022.281445 PMid:35924584 PMCid:PMC9614520
- Miller
RP, Tadagavadi RK, Ramesh G, Reeves WB. Mechanisms of cisplatin
nephrotoxicity. Toxins (Basel). 2010;2(11):2490-2518.
doi:10.3390/toxins2112490 https://doi.org/10.3390/toxins2112490 PMid:22069563 PMCid:PMC3153174
- Fjeldborg P, Helkjer PE, Introduction HE, Cisplatin OF. The Long-Term Effect. 1986;2:2214-2217. https://doi.org/10.1002/1097-0142(19861115)58:10<2214::AID-CNCR2820581009>3.0.CO;2-I PMid:3756770
- Conway
R, Carey JJ. Risk of liver disease in methotrexate treated patients.
World J Hepatol. 2017;9(26):1092-1100. doi:10.4254/wjh.v9.i26.1092 https://doi.org/10.4254/wjh.v9.i26.1092 PMid:28989565 PMCid:PMC5612840
- Wilder
J, Patel K. The clinical utility of FibroScan® as a noninvasive
diagnostic test for liver disease. Medical Devices: Evidence and
Research. 2014;7(1):107-114. doi:10.2147/MDER.S46943 https://doi.org/10.2147/MDER.S46943 PMid:24833926 PMCid:PMC4014361
- Li G zhou, Hu Y hui, Li D yi,
et al. Vincristine-induced peripheral neuropathy: A mini-review.
Neurotoxicology. 2020;81(August):161-171.
doi:10.1016/j.neuro.2020.10.004 https://doi.org/10.1016/j.neuro.2020.10.004 PMid:33053366
- Steensma
DP. Predicting therapy-related myeloid neoplasms-and preventing them?
Lancet Oncol. 2017;18(1):11-13. doi:10.1016/S1470-2045(16)30622-2 https://doi.org/10.1016/S1470-2045(16)30622-2 PMid:27927581
- Chen
Y, Estrov Z, Pierce S, et al. Myeloid neoplasms after breast cancer:
"therapy-related" not an independent poor prognostic factor. Leuk
Lymphoma. 2015 Apr;56(4):1012-9. doi: 10.3109/10428194.2014.946023 https://doi.org/10.3109/10428194.2014.946023 PMid:25048874 PMCid:PMC4326620
- Bertoli
S, Sterin A, Tavitian S, et al. Therapy-related acute myeloid leukemia
following treatment of lymphoid malignancies. Oncotarget. 2016 Dec
27;7(52):85937-85947. doi: 10.18632/oncotarget.13262. https://doi.org/10.18632/oncotarget.13262 PMid:27852053 PMCid:PMC5349887
- Sorror
ML, Maris MB, Storb R, et al. Hematopoietic cell transplantation
(HCT)-specific comorbidity index: A new tool for risk assessment before
allogeneic HCT. Blood. 2005;106(8):2912-2919.
doi:10.1182/blood-2005-05-2004 https://doi.org/10.1182/blood-2005-05-2004 PMid:15994282 PMCid:PMC1895304
- Elborai
Y, Hafez H, Moussa EA, et al. Comparison of toxicity following
different conditioning regimens (busulfan/melphalan and
carboplatin/etoposide/melphalan) for advanced stage neuroblastoma:
Experience of two transplant centers. Pediatr Transplant.
2016;20(2):284-289. doi:10.1111/petr.12638 https://doi.org/10.1111/petr.12638 PMid:26614402
- Socié
G, Rizzo JD. Second solid tumors: Screening and management guidelines
in long-term survivors after allogeneic stem cell transplantation.
Semin Hematol. 2012;49(1):4-9. doi:10.1053/j.seminhematol.2011.10.013 https://doi.org/10.1053/j.seminhematol.2011.10.013 PMid:22221779
- Doney
K, Leisenring W, Linden H. Allogeneic hematopoietic cell
transplantation in patients with a hematologic malignancy and a prior
history of breast cancer. Breast Cancer Res Treat. 2022;194(3):507-516.
doi:10.1007/s10549-022-06658-5 https://doi.org/10.1007/s10549-022-06658-5 PMid:35779160
- Palmieri
R, Montgomery RB, Doney K. Allogeneic stem cell transplantation in
patients with a prior history of prostate cancer. Ann Hematol.
2023;102(2):407-412. doi:10.1007/s00277-022-05041-0 https://doi.org/10.1007/s00277-022-05041-0 PMid:36394580
- Steensma
DP. Predicting therapy-related myeloid neoplasms-and preventing them?
Lancet Oncol. 2017;18(1):11-13. doi:10.1016/S1470-2045(16)30622-2 https://doi.org/10.1016/S1470-2045(16)30622-2 PMid:27927581